Posts Tagged ‘OSNAP’
Tenth Refresh of the Irminger Sea Array
On August 27th, a team of 13 scientists and engineers boarded the R/V Neil Armstrong in Reykjavik, Iceland to head to the Irminger Sea Array. Most of the array’s infrastructure and instrumentation was shipped from Woods Hole Oceanographic Institution (WHOI) in mid-July to Iceland, where it arrived in mid-August. Part of the scientific party traveled to Reykjavik in mid-August to reassemble the moorings and conduct a “burn-in,” a test period for the power, data, telemetry, and instrument systems to ensure everything is operational prior to loading the vessel.
The Irminger Sea Array is in a region with high wind and large surface waves in the North Atlantic and is one of the few places on Earth with deep-water formation that feeds the large-scale thermohaline circulation. Data collected by the Irminger Sea Array are providing critical insights into circulation patterns, ocean processes, and possible climate-induced changes occurring in this important oceanic area.
After an ~ two-day transit (550 nautical miles) to the array site off the tip of Greenland, the team will recover and deploy four moorings and three gliders over the next two and a half weeks. They will conduct CTD (conductivity, temperature, and depth) casts at the deployment/recovery sites and carry out shipboard sampling for field validation of the platforms and sensors that will remain in the water for the next year.
In addition to the recovery and deployment operations, the team will be conducting some CTD calibration casts in support of OSNAP-GDWBC (Overturning in the Subpolar North Atlantic Program-Greenland Deep Western Boundary Current). A participant from the National Oceanic and Atmospheric Administration will also be on board using “Big Eye” binoculars mounted on a forward deck to make observations of marine mammals during the transit and in the Irminger Sea.
[media-caption path="https://oceanobservatories.org/wp-content/uploads/2023/08/Big-eyes.jpg" link="#"]Large, deck-mounted binoculars known as “big eyes” are used for marine mammal observations. NOAA Research Wildlife Biologist Peter Duley will be aboard the R/V Neil Armstrong watching for marine life in the Irminger Sea. Credit: Al Plueddemann ©WHOI.[/media-caption]The Irminger Team will also be testing out some equipment modifications on this deployment. One change is an updated satellite telemetry system. This system would provide higher bandwidth allowing better and quicker data transmission from the global surface mooring potentially saving power, and better remote command and control of the mooring systems. Another change is a revised mounting scheme for the glider optode, which measures dissolved oxygen concentrations in the water column. The new mount may provide better in-air measurements during glider surfacing. The in-air measurements allow scientists to characterize the changing accuracy of the instrument over time.
“It’s always a challenge to get ready for this month-long expedition to this remote, but critical region, but we are ready and eager to get there,” said John Lund, Chief Scientist for Irminger 10. “We are pleased to play a part in collecting data that scientists are using to better understand changes occurring in this region, with implications for both weather and climate.”
The team will reporting regular updates from the field. Bookmark this page so you can follow along on their progress.
Read MoreAtlantic Water Influence on Glacier Retreat
Adapted and condensed by OOI from Snow et al., 2021, doi:/10.1029/2020JC016509
The warming of Atlantic Water along Greenland’s southeast coast has been considered a potential driver of glacier retreat in recent decades. In particular, changes in Atlantic Water circulation may be related to periods of more rapid glacier retreat. Further investigation requires an understanding of the regional circulation. The nearshore East Greenland Coastal Current and the Irminger Current over the continental slope are relatively well studied, but their interactions with circulation further offshore are not clear, in part due to relatively sparse observations prior to establishing the OOI Irminger Sea Array and the Overturning in the Subpolar North Atlantic Program (OSNAP).
[media-caption path="/wp-content/uploads/2022/04/Pioneer-highlight.png" link="#"]Satellite-derived sea surface temperature after adjustment for the Irminger Current (IC; green), Shelf Trough (ShTr; orange), and East Greenland Coastal Current (EGCC; purple). Monthly values (thin lines) are shown for 2000-2018 with 24-month low-passed records overlain. In situ observations from the fjord mouth (290 m: Black) and OOI flanking mooring FLMA (180 m; blue) are shown for comparison.[/media-caption]
In a recent study (Snow et al., 2021) use in-situ mooring data to validate satellite SST records and then use the 19-year satellite record to investigate relationships between glacier melt and Atlantic Water variability. In order to use the satellite records for this purpose, several adjustments must be made, including accounting for cloud and sea ice contamination, eliminating seasonally-varying diurnal biases, and removing the influence of air temperature. This adjusted satellite SST can be compared to in-situ mooring data during a portion of the record. A coastal mooring near the Sermilik Fjord mouth and the OOI Irminger Sea Array provide useful records during 2009-2013 and 2014-2018, respectively (Figure 24). An interesting aspect is that the temperature record from OOI Flanking Mooring A (FLMA) is useful for this purpose even though the measurements are at 180 m depth. This is because the upper ocean is relatively homogeneous in this region, and the mixed layer is deeper than 180 m during much of the year. The authors find that the adjusted satellite SST is consistent with the in-situ records on monthly to interannual time scales (Figure above). This provided the motivation to investigate relationships between the 19 year satellite record and glacier discharge rates.
The study concludes that warmer upper ocean temperatures as far offshore as the OOI Irminger Sea Array were concurrent with increased glacier retreat in the early 2000s, in support of the idea that Atlantic Water circulation plays a role. However, they also note that this influence is not direct, because of substantial variation in how Atlantic Water is diluted as it flows across the shelf towards Sermilik Fjord. The idea that time-varying dilution of Atlantic Water governs the temperature of water reaching the glacier was not previously understood, and resolving such small-scale, time-varying processes is a challenge for models. The authors conclude that with appropriate adjustments, “[satellite] SSTs show promise in application to a wide range of polar oceanography and glaciology questions” and that the method can be generalized to other glacier outflow systems in southeast Greenland to complement relatively sparse in-situ records.
Snow, T., Straneo, F., Holte, J., Grigsby, S., Abdalati, W., & Scambos, T. (2021). More than skin deep: Sea surface temperature as a means of inferring Atlantic Water variability on the southeast Greenland continental shelf near Helheim Glacier. J. Geophys. Res: Oceans, 126, e2020JC016509. https://doi.org/10.1029/2020JC016509.
Read MoreVideos of OOI’s Virtual Booth Sessions at AGU
In case you missed any sessions at OOI’s Virtual Booth at the AGU Fall 2021 meeting, you can watch them here at your leisure:
[embed]https://vimeo.com/657572792[/embed] [embed]https://vimeo.com/657554340[/embed] [embed]https://vimeo.com/657568018[/embed] [embed]https://vimeo.com/657540648[/embed] [embed]https://vimeo.com/657477409[/embed] [embed]https://vimeo.com/657874176[/embed] [embed]https://vimeo.com/657995699[/embed] Read MoreIrminger Array Successfully Turned 8th Time
The Irminger 8 Team successfully wrapped up the eighth turn of the Global Irminger Sea Array on 26 August when the R/V Neil Armstrong docked in Reykjavik, Iceland. After a few days of demobilization, the 10 members of the science party were free to head home after showing proof of a negative COVID test 72 hours before boarding a flight back to the U.S.
Chief Scientist John Lund led the science party of 10 in completing all of the expedition’s objectives. Over the course of 26 days at sea, they recovered four moorings and deployed four new moorings in their place. The team also deployed three gliders—two Open Ocean and one Profiling—and recovered a glider that had been in the water since 2020 and whose battery supply was rapidly depleting.
[media-caption path=”https://oceanobservatories.org/wp-content/uploads/2021/08/Armstrong-and-Iceberg-e1629493552453.jpg” link=”#”]The Irminger Sea presents challenges of high winds, strong waves, and icebergs as shown here with the R/V Neil Armstrong in the foreground. Credit: drone video, Croy Carlin SSSG. [/media-caption]One highlight of the trip was engaging in scientific outreach with a class of fourth graders. The team connected with the students while out on the open ocean via Zoom. The oceanographers aboard the ship each had a chance to share what it’s like being on an oceanographic voyage and explain the purpose of the different instruments and sensors on the arrays. Another highlight of the expedition was the OOI team’s ongoing collaboration with OSNAP (Overturning in the Subpolar North Atlantic Program). While OSNAP participants were not onboard the Armstrong as in the past, their shore-based presence was clearly in evidence. Expert hydrographer Leah McRaven worked with the onboard team to adjust CTD (Conductivity, temperature, depth) sampling to ensure that new CTD equipment was calibrated and sampling properly.
The science team also added a novel twist to the regular shipboard sampling that supports field calibration and validation of the platforms and sensors in the arrays. During Irminger 8, the shipboard team worked with OOI’s onshore data team to make collected CTD data available online in near real-time. As an added bonus, McRaven shared her insights about CTD sampling in regular blog posts here.
The Irminger 8 Team took full advantage of being in this critical ocean region, which is sensitive to climate change. During transit from Woods Hole to the array, off the southeast coast of Greenland, the team deployed surface drifters and ARGO floats for the Greenland Freshwater Project, which is studying the impact of freshwater runoff from Greenland’s melting ice sheet on the North Atlantic and Arctic climate. The team also deployed a biogeochemical ARGO float for the Global Ocean Biogeochemistry Project, and took a series of CTD casts on behalf of OSNAP, to add to long term data collection efforts in this critical region. In addition, the team deployed two RAFOS floats for the Madagascar Basin Project to measure deep water circulation and 15 Sofar Spotter buoys to measure wind, wave, and temperature data.
“In the ideal, science is a collaborative process,” said Chief Scientist John Lund. “During transit time to and from the array, we were able to help our scientific partners get their equipment in the water. The data provided will help advance understanding of this critically important region, which is equally difficult to sample. The region has high winds, large, steep waves, strong currents, icebergs, and consequent equipment icing.”
Given the challenges of the ocean environment at these latitudes, the eighth turn of Irminger Array included equipment improvements. The newly deployed surface moorings included wind turbine modifications to help it withstand strong, volatile winds, and it also incorporated other structural modifications to strengthen the mooring, while easing refurbishment. Similarly, design modifications were made to the subsurface moorings to help ensure consistent, long-term data collection.
The team experienced some of these challenges of high winds and strong waves while on the cruise, but the rough conditions were compensated by the gorgeous scenery of the region. Added Lund, “One afternoon, the sun came out as the ship transited further up Prince Christian Sound. Everyone was awed by the beauty of the landscape. We saw glaciers, icebergs and the occasional whale.”
Prior to leaving the Sound, the team secured all the items for the transit to Reykjavik, the demobilization of the ship, and finally the journey home to Woods Hole.
Read More
Everything You Need to Know about CTD data
In August 2021, expert hydrographer, Leah McRaven (PO WHOI) from the US OSNAP (Overturning in the Subpolar North Atlantic Program) team, worked with the OOI team members aboard the R/V Neil Armstrong for the eighth turn of the Global Irminger Sea Array to support collection of an optimized hydrographic data product. A truly novel aspect of this collaboration was the near real-time sharing of OOI shipboard CTD data with the public. McRaven also shared her reports while the cruise is underway. In doing so, she provided a detailed explanation of the process of ensuring that CTD profiles are accurate and useable for future research use.
We share her blogs below. For archival purposes, they will also be available on the Community Tools and Datasets page.
BLOGPOST #4 (September 13, 2021)
Another OOI cruise is in the books! Now that things have wrapped up and I’ve had a chance to dig into the data a bit more thoroughly, how did we do? In my previous post I reported that the Irminger 8 CTD data looked to be very promising, but I like to include one more step before recommending data to be used for science: carefully considering salinity bottle data.
Salinity bottle data can be used in many ways to support a particular scientific objective or research question. The two that I’ve become most familiar with are 1) to support the analysis of additional bottle samples (e.g. dissolved oxygen) and 2) to provide an additional assessment and calibration of the CTD conductivity sensors. Both applications are necessary when researchers require salinity values more accurate than what CTD sensors are able to provide. However, even if this is not required, it can help ensure that users receive data that are reasonably within manufacturer specifications.
I find it easiest to consider the GO-SHIP approach to bottle data first. Using ship-based hydrography, GO-SHIP provides approximately decadal resolution of the changes in inventories of heat, freshwater, carbon, oxygen, nutrients, and transient tracers, covering the ocean basins with global measurements of the highest required accuracy to detect these changes. For a program like this, 36 salinity samples are taken every CTD station in order to provide an extremely accurate and precise calibration for the CTD sensors. Interestingly, the Irminger OOI array is bracketed by three GO-SHIP repeat transects. While GO-SHIP provides invaluable measurements, drawing a large number of samples can be expensive and time consuming. Additionally, measurements occur on a decadal timescale, so there is a lot of the picture we miss.
One of the research programs that aims to provide a higher temporal and special resolution picture of the North Atlantic is OSNAP. This program has several scientific objectives, but generally aims to quantify intra-seasonal to interannual variability of the Atlantic Meridional Overturning Circulation(AMOC) in the subpolar Atlantic. This includes a focus on heat and freshwater fluxes, pathways of currents throughout the region, and air-sea interaction, all of which require highly calibrated data products. In order to accomplish this, PIs from the program need to be able to consistently merge their shipboard and moored data products for cohesive and accurate quantification of parameters. Because much of the variability being studied is so large, researchers do not necessarily need salinity accuracies at the level of GO-SHIP, but they do need to use salinity bottle samples to ensure that CTD casts are at the very least within manufacturer specifications.
In the end, no one approach to hydrographic sampling is necessarily better than another. What is important is the delicate balance of resources while at sea that best support the scientific objectives. For both OSNAP and OOI, where the primary work at sea is focused on servicing moorings, the resources for a GO-SHIP approach to sample bottle collection is simply not feasible. However, one very key feature of the OOI CTD data is that they are collected annually, while ONSAP data are collected every two years, and GO-SHIP data are collected every ten years. Hence, OOI is able to fill in some of the temporal data gaps in the region and greatly bolster many of the international programs working in the region.
This year OOI collaborated with numerous PIs and representatives from research programs that operate in the Irminger Sea region to produce a more optimized CTD and bottle sampling strategy that better complements goals similar to GO-SHIP, as well as several additional objectives from international programs, such as OSNAP. The goal of this updated plan was to provide OOI data end users and collaborators with data that are more appropriate for CTD, mooring, glider, and float instrumentation calibration purposes. In particular, the update included increased sampling of the deep ocean. Such data are critical in the Irminger Sea region due to the uniquely large variability of temperature, salinity, and chemical properties throughout the shallow and intermediate depths of the water column. Deeper CTD and bottle data will allow all end users to more carefully reference their scientific findings to more stable water masses and allow for better intercomparison with other available datasets, such as the available GO-SHIP and OSNAP data from the region.
The majority of methods that I use when considering salinity bottle data have been adapted from GO-SHIP and NOAA/PMEL. In particular, many of the cruises I work with, including OOI, often have far fewer bottle samples than recommended by GO-SHIP or PMEL methods. This isn’t necessarily bad, since we don’t need to achieve the same goals as those programs, however, great care in adapting methods does need to be considered (and I encourage you to reach out if this is something you have an interest in). So, with the improved OOI sampling scheme, what are the potential benefits to CTD data quality?
More strategically planned salinity bottle sample collection allows users to:
- Decide if data from primary or secondary sensors are more physically consistent
- Identify times when CTD contamination was not obvious
- Assess manufacturer sensor calibrations
- Potentially provide a post-cruise calibration
In the case of the Irminger 8 cruise, I see that all four uses of salinity bottle data are possible, which will make a lot of collaborators very happy! Starting with Figure 1, we can see a summary of CTD and bottle sample salinity differences as a function of pressure for both the primary and secondary sensors. As a rule of thumb, the average offset of these differences can be considered an estimate of sensor accuracy, and the spread, or standard deviation, can be considered an estimate of sensor precision. While the data have a fairly large spread to the eye, the standard deviations (indicated by the dashed lines) are placing the spread for each sensor within what we expect from the manufacturer precision. The striking result from this figure is that before using the bottle data to further calibrate the data, we see that the primary sensor had a higher accuracy than the secondary sensor. In going back to the Seabird Electronics calibration reports for the primary and secondary sensors (available via the OOI website), I noted that the calibrations for each sensor was a bit older than what we normally work with (last performed in May 2019). Additionally, the secondary sensor had a larger correction at its time of manufacturer calibration than the primary. This is corroborated by the differing sensor accuracies as determined by the bottle data. Lastly, while there are a few spurious differences shown, on average there doesn’t look to be any CTD and bottle differences due to factors other than expected calibration drifts.
Figure 1
In order to apply a calibration based on the bottle data to the CTD data, I first QC’d the bottle data and then followed methods described in the GO-SHIP manual. There are several sources of error that can contribute to incorrect salinity bottle values, ranging from poor sample collection technique to an accidental salt crystal dropping into a sample just before being run on a salinometer. This is why all methods of CTD calibration using bottle data stress the importance of using many bottle values in a statistical grouping. However, sometimes there are “fly-away” values that are so far gone they don’t contribute meaningful information to the statistics, and in those cases I simply disregard those values. As a reference, for the Irminger 8 cruise I threw out 9 of the ~125 salinity samples collected before proceeding with calibration methods. Note that within the methods described in the above documentation, systematics approaches are used to further control for outlier or “bad” bottle values.
Figure 2
Since the majority of CTD stations for OOI are performed close to one another (and consequently in similar water masses), I grouped all stations together to characterize sensor errors. The resulting fits produced primary and secondary sensor calibrations that allow for more meaningful comparison of data with other programs. Figure 2 shows how primary and secondary data compare before and after bottle calibrations have been applied. Post calibration, primary and secondary sensors now agree more closely in terms of their differences. Similarly, Figure 3 summarizes data before and after calibration in temperature-salinity space, providing visual context for the magnitude of bottle calibration. Many folks working with CTD data would say that this is a rather small adjustment!
Figure 3
Figure 4
However, Figure 4 shows a comparison of the bottle-calibrated OOI data with nearby OSNAP CTD profiles from 2020. The results here are extremely important as OSNAP currently has moorings deployed near the OOI array and the OOI CTD profiles provide a midway calibration point for the moored instrumentation that is currently deployed for two years. These midway calibration CTD casts are critical in providing information on moored sensor drift and biofouling in a region where there has been a slow freshening of deep water (colder than 2.5 ºC) throughout the duration of these programs. Quantifying the rate of freshening is one of the objectives that OSNAP focuses on, but it is nearly impossible without high-quality CTD data for comparison. Figure 4 demonstrates that the freshening trend has continued from 200 to 2021 and that the bottle-calibrated OOI CTD data will be critical for interpretation of moored data.
Finally, for those interested in the salinity-calibrated CTD dataset, please be in touch (lmcraven@whoi.edu). A more detailed summary of the calibration applied can be found in my CTD calibration report here.
BLOGPOST #3 (August 18, 2021)
Irminger 8 science operations are now fully underway, which means the stream of CTD data is coming in hot (actually the ocean temps are very cold)! So far, CTD stations 4 through 11 correspond to work performed near the Irminger OOI array location. I spent the weekend and past couple of days paying close attention to these initial stations. Here’s an update on how things look so far.
One of the concerns this year is that the R/V Neil Armstrong is using a new CTD unit and sensor suite (new to the ship, not purchased new). Any time a ship’s instrumentation setup changes, it’s a very good idea to keep a close eye on things as changes naturally mean there’s more room for human error. What better way to talk about this than to share my own mistakes in a public blog! When I first downloaded and processed the OOI Irmginer 8 (AR60) CTD data from near the Irminger OOI array location, I became very worried…
When I compared the Irminger 8 CTD data with three cruises from the same location last year, I was seeing very confusing and unphysical data in my plots. I was using Seabird CTD processing routines in the “SBE Data Processing” software (see https://www.seabird.com/software) that I had used for previous OOI Irminger cruises as a preliminary set of scripts, so I was confident that something strange with the CTD was going on. I immediately pinged folks on the ship to ask if there was anything that they could tell was strange on their side of things. Keep in mind, this OOI cruise focuses more on mooring work with only a handful of CTDs to support all additional hydrographic work, so any time there is a potential issue with the CTD data we want to address it as soon as possible. I started digging into things a bit more, and realized that I had made a mistake.
Within the SBE processing routines, there is a module called “Align CTD”. As stated in the software manual: “Align CTD aligns parameter data in time, relative to pressure. This ensures that calculations of salinity, dissolved oxygen concentration, and other parameters are made using measurements from the same parcel of water. Typically, Align CTD is used to align temperature, conductivity, and oxygen measurements relative to pressure.” When working in areas where temperature and salinity change rapidly with pressure (depth), this module can be very important (and I encourage you to read through its documentation). As you’ll see below, the OOI Irmginer Sea Array region can see some extremely impressive temperature and salinity gradients. This has to do with the introduction of very cold and very fresh waters from near the coast of Greenland, together with the complect oceanic circulation dynamics of the region. Based on data collected on the old CTD installed on the Armstrong, I had determined that advancing conductivity by 0.5 seconds produced a more physically meaningful trace of calculated salinity.
While 0.5 seconds doesn’t seem large, it’s important to remember that most shipboard CTD packages are lowered at the SBE-recommended speed of 1 meter/second. Depending on how suddenly properties change as the CTD is lowered through the water, this magnitude of adjustment may seriously mess things up if it’s not the correct adjustment. In the case of the CTD system currently installed on the Armstrong, I’m finding that very little adjustment to conductivity is needed. There are many reasons as to why this value will change – from CTD to CTD, cruise to cruise, and even throughout a long cruise. The major factor is the speed at which water flows through the CTD plumbing and sensors and how far the pressure, temperature, and conductivity sensors are from each other in the plumbed line. Water flow is controlled by many things including CTD pump performance, contamination in the CTD plumbing, kinks in the CTD pluming lines, etc. (for more information, start here: https://www.go-ship.org/Manual/McTaggart_et_al_CTD.pdf and here: file:///Users/leah/Downloads/manual-Seassoft_DataProcessing_7.26.8-3.pdf). Note that the SBE data processing manual provides great tips on how to choose values for the Align CTD module.
Below is a figure that summarizes impact on my processed data before and after my mistake. This is a fun figure as it compares CTD data from four cruises that all completed CTDs near the OOI site: the 2020 OSNAP Cape Farewell cruise (AR45), the 2020 OOI Irminger 7 cruise (AR46), a 2020 cruise on R/V Pelagia from the Netherlands Institute for Sea Research, and stations 5-7 of the 2021 OOI Irminger 8 cruise (AR60). I’m plotting the data in what is called temperature-salinity space. This allows scientists to consider water properties while being mindful of ocean density, which as mentioned in the last post, should always increase with depth. I include contours indicating temperature and salinity values that correspond to lines of constant density (in this case I am using potential density referenced to the surface). For data to be physically consistent, we expect that the CTD traces never loop back across any of the density contours. These figures are also incredibly useful as the previous three cruises in the region give us some understanding of what to expect from repeated measurements near the Irminger OOI array.
The plot on the left shows the data processed with a conductivity advance of 0.5 sec. As you can see, the CTD traces appear much noisier than the other datasets, and contain many crossings of the density contours (i.e., density inversions). The plot on the right shows data that are smoother and less problematic in terms of density. You may also note that in the right plot, the AR60 traces are a bit shifted to the left in salinity (i.e. fresher or lower salinity values) when compared to the other datasets. This is because I am plotting bottle-calibrated CTD data from the other three cruises. Just as I type this, I’ve been given word that the shipboard hydrographer has begun analyzing salinity water sample data for Irminger 8. These bottle data are critical for applying a final adjustment to CTD salinity data and I’ll talk more about this in a future post.
For now, I’m happy to report that the data look physically consistent! For completeness, I include the core CTD parameter difference plots from stations 4 through 11 (CTDs completed near the array thus far). CTD difference plots are described in my previous post. All checks out from where I am sitting so far. Thanks to the CTD watch standers and shipboard technicians for working so hard and taking good care of the system while the cruise is underway!
BLOGPOST #2 (August 10, 2021)
For this post I’d like to introduce some of the tools that folks can use to identify CTD issues and sensor health while at sea. Most of what I’ll be discussing here is specific to the SBE911 system that is commonly used on UNOLS vessels; however, a lot of these topics are relevant to other types of profiling CTD systems.
There are several end case users of CTD data within science. These include people who perform CTDs along a track and complete what we call a hydrographic section (useful in studying ocean currents and water masses); those who perform CTDs at the same location year after year to look for changes; people who use CTDs to calibrate instrumentation on other platforms (moorings, gliders, AUVs, etc.); and those who use the CTD to collect seawater for laboratory analysis (collected samples can be used to further analyze physical, chemical, biological, and even geological properties!). For each of these CTD uses, a core set of CTD parameters are needed.
Core CTD parameters include pressure, temperature, and conductivity. Conductivity is used together with pressure and temperature measurements to derive salinity. These three variables are needed to give users the critical information of depth and density in which water samples are collected, and support the calculation of additional variables. For example, ocean pressure, temperature, and salinity together with a voltage from an oxygen sensor are needed to derive a value for dissolved oxygen. In addition to core CTD parameters, it is very common to add dissolved oxygen, fluorescence, turbidity, and photosynthetically active radiation (PAR) sensors to a shipboard CTD unit. Each of these additional measurements have errors that must be propagated from the core CTD measurements – creating a rather complex system to navigate when trying to understand the final accuracy of a given measurement.
For each CTD data application, varying degrees of accuracy are needed from the measured CTD parameters to accomplish the scientific objective at hand… And this is where a lot of folks get into trouble! For a first example, consider someone who would like to calibrate a nitrate sensor that is deployed for a year on a mooring using water samples collected from the CTD. For a second example, consider someone who is interested in the changing dissolved oxygen content of deep Atlantic Meridional Overturning Circulation waters. In both cases, the core CTD parameters are critical. In the first example, this person needs to know the pressure and ocean density at the exact location their water samples are collected during a CTD cast so they can correctly associate analyzed water sample values with the correct position of the sensor on the mooring. However, in the second example, this person may need to use both salinity and oxygen samples to improve the accuracy and precision of CTD measurements so that their final data product will be sensitive enough to resolve small, but potentially critical, changes in the ocean.The most important take away here (CTD soapbox moment!) is that even if end users are not specifically interested in studying physical oceanographic parameters, they still need tools to verify that 1) the core CTD measurements are of high enough quality for use in their application and 2) that there are no unnecessary errors from the core measurements that are impacting their ability to address their scientific objective.
This is the first reason why I heavily encourage all CTD end users to become familiar not only with the accuracy of their particular measured parameter, but also the core CTD parameters. The second reason is that core CTD parameters are particularly useful in diagnosing early warning signs of CTD problems. Most shipboard systems install primary and secondary temperature and conductivity sensors on their CTDs, which provide an opportunity for in-situ sensor comparison. Additionally, calculated seawater density is particularly useful as it is one of the few properties we can make a strong assumption about – it should always increase with pressure. The density of seawater is determined by pressure, temperature, and salinity (conductivity), hence any time one or some of these recorded values is suspect, non-physical density “inversions” or “noise” may appear in the data record.
Below are two figures that can be very helpful in diagnosing CTD problems. In these examples, I am using the Irminger 8 (AR60-01) deep test cast, which took place late Sunday evening, August 8th. Figure 1 shows difference plots of the two sensor pairs (temperature and conductivity). Each panel includes vertical dashed lines indicating expected manufacturer agreement ranges (see sensor specification sheets datasheet-04-Jun15.pdf and datasheet-03plus-May15.pdf). The values shown are, ±(2 x 0.001 ºC) and ±(2 x 0.003 mS/cm) for temperature and conductivity sensors, respectively (note that 0.003 mS/cm is close to 0.003 psu for reasonable temperature ranges). In general, sensor differences should fall within, or very close to, this range when calibrated by the manufacturer within the past year. The rule can be relaxed in the upper water column, however, differences between sensors deeper than approximately 500 m that consistently fall outside of this range indicate problematic sensor drift or contamination. Figure 2 shows the calculated seawater density profile using the primary sensors. Consistent density inversions larger than ~0.1 kg/m3 also indicate problematic sensor drift or contamination. When creating such figures, always look at the downcast and upcast (skipped here for the sake of brevity). The upcast will look a bit worse than the downcast (I encourage you to read about why), but those data are extremely important to anyone collecting water samples!
Figure 1
Figure 2
So, what can these plots tell us about the CTD system implemented on the Irminger 8 cruise so far? Figure 1 demonstrates an overall acceptable level of agreement between the sensor pairs. The particular sensors in use right now have calibrations older than one year, so this level of agreement is actually quite good. Figure 2 is also rather promising in showing a density profile that is continuously increasing. If you’re being picky (like me), you may notice that there are some small density inversions between roughly 200-500 m. After taking a closer look, I noted that the salinity profile indicates that there are some rather impressive salinity intrusions evident in the upper 500 meters (I encourage you to download data from cast 2 and verify!). This is normal for the Labrador Sea region where the cast took place (lots of melting ice nearby) and will naturally create a bit more “noise” in these plots. So, I’m not very concerned by this.
Now what do these plots look like when there’s a problem? There unfortunately isn’t one simple answer for this (I’ve been doing this for over ten years and am still learning subtle ways CTDs show problems!), but I’ll share two examples of when something was clearly wrong. The first example is from the Irminger 7 cruise (AR35-05). Figure 3 and Figure 4 show our two plots for stations 1-13 of the Irminger 7 cruise. Figure 3 shows a suspiciously large offset (well outside of the general threshold we expect in the conductivity differences) and incredibly noisy differences in both conductivity and temperature. Similarly, Figure 5 shows consistent and large density inversions for some of these casts. Several of the casts shown in Figures 4 and 5 were so bad that there are no usable profiles as far as scientific objectives are concerned. Luckily, however, there were a few casts in the set that could be corrected with water sample data (I’ll talk more about this later). Data loss is something that does happen while at sea, and the Irminger Sea in particular is an incredibly harsh environment to work in. However, if folks are diligent in creating these plots while at sea, the hope is that we can minimize time and data loss while striving for the highest quality data possible.
Figure 3
Figure 4
For my last example, I provide a quick reference guide for how core CTD parameter issues may look on a Seabird CTD Real-Time Data Acquisition Software (Seasave) screen. The reason for this is that a lot of people don’t have time to create fancy plots while at sea, so it’s helpful to know how to approach monitoring while watching the data come in. Follow the link here to download a one-page pdf that can be displayed next to your CTD acquisition computer.
BLOG POST #1 (August 2, 2021)
[caption id="attachment_21736" align="aligncenter" width="2560"]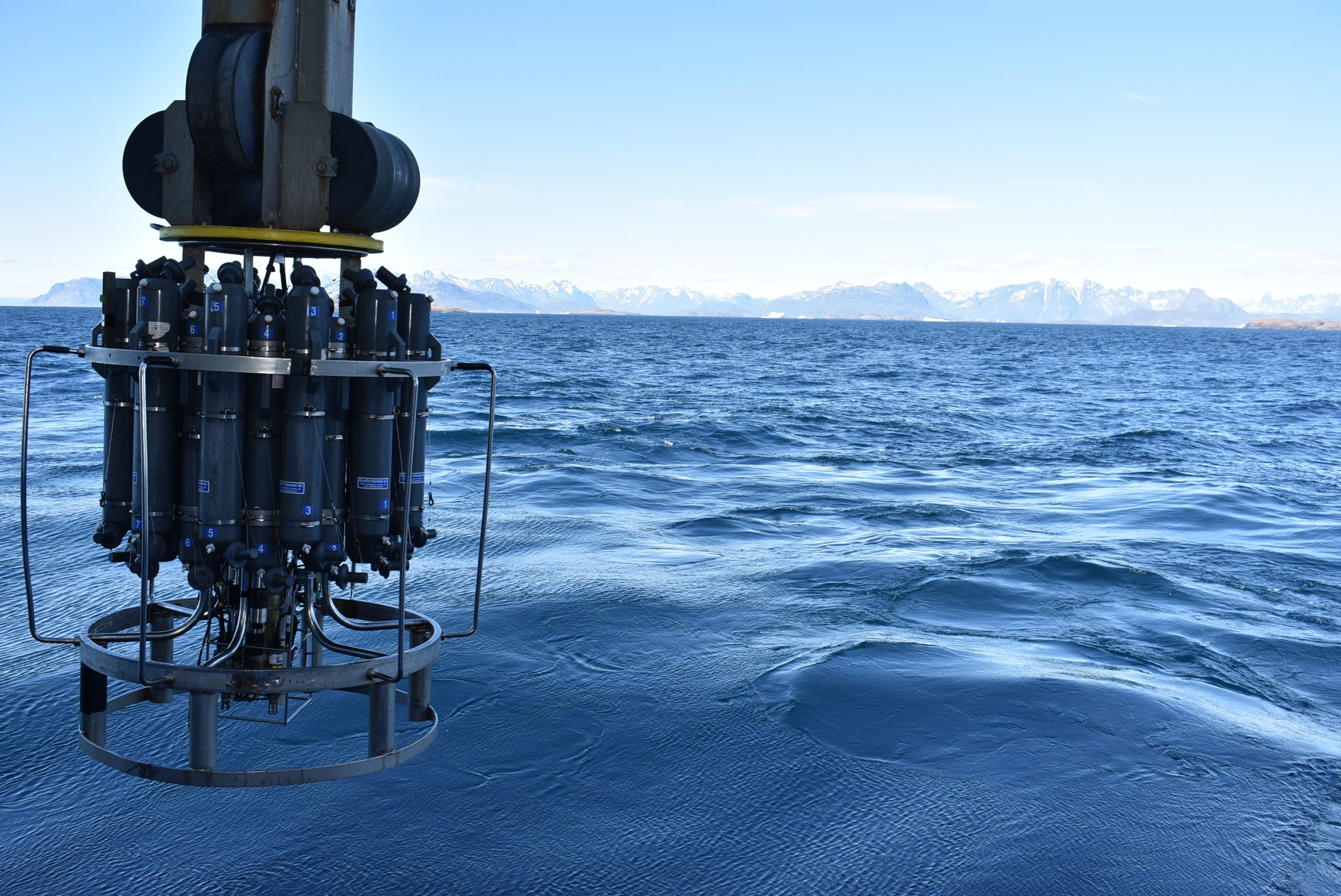
Hello folks and welcome to the Irminger 8 CTD blog! As the cruise progresses, tune in here for updates on Irminger 8 CTD data quality as well as tips on how best to approach using OOI CTD data. I plan to keep this information inclusive for folks with varying levels of experience with shipboard CTD data – from beginner to expert! If you have any questions about CTD data, feel free to send me an email (lmcraven@whoi.edu) and I’ll do my best to help. For this first post, I would like to summarize some important resources available to the community that will greatly help with CTD data acquisition and processing.
CTDs have been around for a while, which on the surface makes them a bit less interesting than many of the new exciting technologies used at sea. The fact remains that the CTD produces some of the most accurate and reliable measurements of our ocean’s physical, chemical, and biological parameters. Aside from being very useful on their own, CTD data serve as a standard by which researchers can compare and validate sensor performance from other platforms: gliders, floats, moorings, etc. Sensor comparison is particularly important for instruments that are deployed in the ocean for a long time (as is the case for OOI assets) as it is normal for sensors to drift due to environmental exposure and biological activity. As it turns out, CTD data provide a backbone for all OOI objectives.
However, just because CTDs have been performed for decades, we can’t always assume that that collection of quality data is straightforward. For example, one of the unique challenges of collecting CTD data near the OOI Irminger site and Greenland region is that there is an elevated level of biological activity throughout the year. While biological activity is exciting for many researchers, it can clog instrument plumbing, build up on sensors, and just be plain annoying to watch out for. CTDs utilized in the Irminger Sea are also subject to extreme conditions such as cold windchills and rough sea state (Cape Farewell is actually the windiest place on the ocean’s surface!), leading to the potential for accelerated sensor drift and the need to send sensors back to manufacturers for more regular servicing and calibration. As one can imagine, there are a lot of potential sources of error when simply considering the environment that OOI Irminger CTD data are collected in.
To help combat some of these potential sources of error, I’ll be picking apart CTD and bottle data cast by cast to look for evidence of CTD problems during the Irminger 8 cruise. But before we can talk about unique sources of CTD data errors, it’s helpful to remember errors that can become systematic throughout the entire data arc: from instrument care, to acquisition, to data processing, and to final data application. Improving our awareness of these issues will allow all CTD data users the opportunity for more meaningful data interpretation. So before I move forward, I thought it would be important to share some of my favorite resources available on community-recommended CTD practices. I encourage folks to comb through these resources and find what might be most appropriate for your respective research objectives.
Recommended CTD resources are provided here.
Read More
Shipboard CTD Data in Near-real Time from Irminger 8
In August, members of the OOI team aboard the R/V Neil Armstrong for the eighth turn of the Global Irminger Sea Array and members of OSNAP (Overturning in the Subpolar North Atlantic Program) onshore are working together to make near-real time shipboard CTD data available here.
[button link="https://oceanobservatories.org/community-tools/"] READ NEAR REAL-TIME CTD DATA REPORT BLOGS DURING CRUISE [/button]
The OOI shipboard team is working directly with an onshore expert hydrographer, Leah McRaven (PO WHOI), from the US OSNAP team to support collection of an optimized hydrographic data product. This collaboration is supporting the OOI team through the cruise planning stages, during the cruise, and during initial data processing stages. In the end, both teams aim to document the process of collecting thoroughly vetted data from the shipboard CTD (conductivity, temperature, depth) system.
A special feature of this collaboration is the near real-time sharing of OOI shipboard CTD data with the public. Interested parties have access to the same CTD profiles that McRaven will be reviewing. Additionally, McRaven will share brief reports online while the cruise is underway.
The hydrographic data collection facilitated by OOI on the Irminger Sea cruise will bolster not only OOI end users, but also supports international oceanographic research projects, including OSNAP, AMOC (Atlantic Meridional Overturning Circulation) and BGC-ARGO (BioGeoChemical Array for Real-time Geostrophic Oceanography).
“We hope sharing this data will present an opportunity for OOI end users to learn more about working with oceanographic data as well as good data practices,” said McRaven.
Al Plueddemann, PI of OOI’s Coastal and Global Scale nodes, which includes the Global Irminger Array, added “This is a great example of a cross-project collaboration that expands the visibility of OOI data over the short-term and improves its quality for integration into long-term research projects like OSNAP.”
Read MoreA Collaborative Month in the Irminger Sea
A team of 10 Woods Hole Oceanographic Institution scientists, who spent the month of August aboard the RV Neil Armstrong, arrived at home port in Woods Hole on 4 September, having successfully skirted Hurricane Laura as she headed in their direction. The bumpy ride home capped the successful deployment of all OOI Irminger Sea Array moorings in sometimes turbulent seas.
While onsite at the array, the team successfully met all of its mission objectives by recovering and deploying four moorings and deploying two gliders. One glider transits the individual moorings, which are spaced approximately 20 km apart, while the second glider samples the upper 200-meters of the ocean above the centrally located hybrid profiler mooring, which measures the remainder of the 2800-m water column. A third glider was recovered soon after deployment because it had a microleak. The team also conducted CTD casts at each of the moorings, which measure onsite temperature, salinity, and oxygen conditions and validate data being collected and sent to shore by the array.
“The Irminger Sea array presents both unique opportunities and challenges for reporting ocean data,“ explained Sebastien Bigorre, who served as chief scientist on the Irminger Seven expedition. “It is located in a remote area of the North Atlantic with high wind and large surface waves, which present operational challenges. The area is also of great interest for scientists and society because of the intense exchange of energy and gases between the atmosphere and the ocean. The ocean there captures heat and carbon dioxide from the atmosphere, thus it is an important component of the climate system. It is also a region of high biological productivity, making it an important fishery. Recent studies have shown that the data collected at the Irminger array are essential to correctly describe the ocean circulation of the North Atlantic.”
It is an eight-day transit from Woods Hole to the Irminger Sea Array and another eight-day transit to return to home port. To maximize the use of ship time, the Irminger Sea Array Team shared ship space and mission time with scientists from OSNAP (Overturning of the Subpolar North Atlantic Program). OSNAP is seeking to provide a continuous record of the horizontal transport of heat, mass, and freshwater in the subpolar North Atlantic, and is complemented by the much longer-term records of water-column properties and air-sea transfer of momentum, heat, and moisture that are provided by the OOI Irminger Sea Array. Once on site, the expedition started with deployment of OOI moorings and gliders, switched its focus to recovery and re-deployment of OSNAP moorings, before finishing with the recovery of the previous year OOI Irminger Sea moorings.
“Our partnership with OSNAP is an example of how we try to maximize our resources for scientific research, from cruise planning, to operations at sea. During transits, we test and triple check our equipment to ensure that comes deployment day, everything goes as smooth as possible. On site, we coordinate operations to accommodate for weather conditions or to optimize shared equipment or personnel. When there is a lull in scientific activities, we plan for the ship’s instrumentation to collect data that is relevant to our scientific objectives, so every hour of the cruise is used to its full potential,” added Bigorre.
The following images show the many tasks undertaken during the month-long expedition:
[media-caption type="image" class="external" path="https://oceanobservatories.org/wp-content/uploads/2020/09/IMG_4208-scaled.jpg" alt="Irminger 7 masks" link="#"]OOI Irminger Sea cruise participants James Kuo (foreground), Jennifer Batryn, and Collin Dobson demonstrate proper social distancing and PPE use on the deck of the R/V Neil Armstrong during departure from the Woods Hole Oceanographic Institution (WHOI) dock Sunday 9 August. Photo credit: Rebecca Travis©Woods Hole Oceanographic Institution.[/media-caption] [media-caption type="image" class="external" path="https://oceanobservatories.org/wp-content/uploads/2020/09/IMG_4215-scaled.jpg" alt="Armstrong awaiting departure" link="#"]The R/V Neil Armstrong is loaded with crew and equipment and ready to depart for the month-long expedition to the Irminger Sea Array. Photo credit: Rebecca Travis©Woods Hole Oceanographic Institution.[/media-caption] [media-caption type="image" class="external" path="https://oceanobservatories.org/wp-content/uploads/2020/09/Screen-Shot-2020-09-14-at-4.56.05-PM.png" alt="Drone overhead" link="#"]A place for everything, everything in its place. Aerial view of the R/V Neil Armstrong deck with equipment loaded for the OOI Irminger Sea Array service cruise. Photo credit: James Kuo©Woods Hole Oceanographic Institution.[/media-caption] [media-caption type="image" class="external" path="https://oceanobservatories.org/wp-content/uploads/2020/09/Glider-with-mask-scaled.jpg" alt="Glider with mask" link="#"]Even the gliders took precautions for the Irminger Sea Expedition! (The tape was removed before deployment). Photo credit: Diana Wickman©Woods Hole Oceanographic Institution .[/media-caption] [media-caption type="image" class="external" path="https://oceanobservatories.org/wp-content/uploads/2020/09/IMG_2081-scaled.jpg" alt="Off stern" link="#"]Global Surface Mooring loaded on the R/V Neil Armstrong deck. It replaced a mooring recovered at the site. Photo credit: James Kuo©Woods Hole Oceanographic Institution.[/media-caption] [media-caption type="image" class="external" path="https://oceanobservatories.org/wp-content/uploads/2020/09/IR7_Dobson_lab-scaled.jpg" alt="Collin in lab" link="#"]Engineer Collin Dobson performs function checks on OOI gliders in the lab of the R/V Neil Armstrong during the transit out to the OOI Irminger Sea array. Photo credit: Jennifer Batryn©Woods Hole Oceanographic Institution.[/media-caption] [media-caption type="image" class="external" path="https://oceanobservatories.org/wp-content/uploads/2020/09/IR7_glider_prep-scaled.jpg" alt="Glider prep" link="#"]Two OOI gliders sit in the lab of the R/V Neil Armstrong during the transit out to the Irminger Sea array. The location of the glider oxygen sensors (blue housings forward of the tail fin) was modified so the sensor is exposed to the air when the glider surfaces. Photo credit:Jennifer Batryn©Woods Hole Oceanographic Institution.[/media-caption] [media-caption type="image" class="external" path="https://oceanobservatories.org/wp-content/uploads/2020/09/20200817_101902.cam3_.jpg" alt="Buoy camera" link="#"]Eyes at sea. This image was captured during the Irminger Global Surface Mooring deployment 17 August 2020 by a camera on the buoy shortly after the buoy was lowered into the water. The camera normally helps operators monitor ice buildup and storm conditions, but on that day it turned its lens on the action aboard the R/V Neil Armstrong. Photo credit: Buoy camera©Woods Hole Oceanographic Institution.[/media-caption] [media-caption type="image" class="external" path="https://oceanobservatories.org/wp-content/uploads/2020/09/SUMOsplice_NicoLlanos_20200811.jpg" alt="Nico splicing" link="#"]Nico Llanos splices lines together, in preparation for the OOI Global Surface Mooring deployment. The surface mooring will be deployed in almost 3,000 m (1.8 mile) of water off of Greenland. Together, the nylon and Colmega add up to almost one mile of rope line, and provide the bottom part of the mooring above its anchor. Photo credit: Heather Furey©Woods Hole Oceanographic Institution .[/media-caption] [media-caption type="image" class="external" path="https://oceanobservatories.org/wp-content/uploads/2020/09/whiteboard_AR46_20200811-1-scaled.jpg" alt="Whiteboard" link="#"]Just like on land, a whiteboard serves as a notice of ongoing and completed activities aboard the R/V Neil Armstrong during the Summer 2020 Irminger Sea month-long expedition. Photo credit: Heather Furey©Woods Hole Oceanographic Institution .[/media-caption] [media-caption type="image" class="external" path="https://oceanobservatories.org/wp-content/uploads/2020/09/IR7_Argo_float.jpeg" alt="Argo float" link="#"]Research Specialist Heather Furey prepares an Argo float for deployment off the stern of the R/V Neil Armstrong. The yellow straps are used to deploy the float while it is still in the box. The cardboard biodegrades in the water and releases the float. Photo credit: Jennifer Batryn©Woods Hole Oceanographic Institution.[/media-caption] [media-caption type="image" class="external" path="https://oceanobservatories.org/wp-content/uploads/2020/09/DSC_0403-scaled.jpg" alt="James Kuo" link="#"]OOI Engineer James Kuo checks the inductive communications on the Irminger Sea Flanking Mooring B during deployment. Most of the instruments on this subsurface mooring transmit data to the mooring controller inductively. The data is then sent acoustically to OOI Gliders which transmit the data to shore via satellite. Photo Credit: Jennifer Batryn©Woods Hole Oceanographic Institution.[/media-caption] [media-caption type="image" class="external" path="https://oceanobservatories.org/wp-content/uploads/2020/09/DSC_0908-1-scaled.jpg" alt="McClane Profiler" link="#"]The OOI team at the Irminger Sea Array deploying the Profiler Mooring. The yellow McLane Moored Profiler with a suite of science instruments is carefully lowered into the water. It will measure water properties including temperature, salinity, fluorescence, dissolved oxygen and water velocity. Photo credit: Jennifer Batryn©Woods Hole Oceanographic Institution.[/media-caption] [media-caption type="image" class="external" path="https://oceanobservatories.org/wp-content/uploads/2020/09/DSC_0012-scaled.jpg" alt="Profiler off stern" link="#"]The OOI Irminger Sea Hybrid Profiler Mooring is deployed top-first and trails behind the ship. Once the ship is at the desired location, the anchor is slid off the back deck, making quite a splash as it falls to the seafloor, pulling the mooring into place. Photo credit: Jennifer Batryn©Woods Hole Oceanographic Institution.[/media-caption] [media-caption type="image" class="external" path="https://oceanobservatories.org/wp-content/uploads/2020/09/Irminger-Sea-Posting--scaled.jpg" alt="Group shot" link="#"]The OOI and OSNAP science team poses on the back deck of the R/V Neil Armstrong on 27 August. 2020, after completing operations at the Irminger Sea Array. Using the last hours of good weather, equipment was secured before the eight-day voyage back to Woods Hole. Photo: Michael Sessa©Woods Hole Oceanographic Institution.[/media-caption] [media-caption type="image" class="external" path="https://oceanobservatories.org/wp-content/uploads/2020/09/northernlights_dobson-scaled.jpeg" alt="Northern lights" link="#"]One of the advantages of going to the OOI Irminger Sea Array is the opportunity to see the northern lights (Aurora borealis).This photo was taken as the team transited home through the Labrador Sea. What a great reward for all of the hard work put in to have a successful cruise! Photo credit: Collin Dobson©Woods Hole Oceanographic Institution.[/media-caption] Read More