RAPID: Multiplexed Distributed Acoustic Sensing (DAS) at the Ocean Observatory Initiative (OOI) Regional Cabled Array (RCA)
[feature]DOI CITATION GUIDANCE Please cite the data in references as: Lipovsky, B., Williams, E., & Ocean Observatories Initiative. (2024). RAPID: Multiplexed Distributed Acoustic Sensing (DAS) at the Ocean Observatory Initiative (OOI) Regional Cabled Array (RCA) [Data set]. Ocean Observatories Initiative. https://doi.org/10.58046/4WEF-A282.[/feature]
[media-caption path="/wp-content/uploads/2024/06/Live-DAS-Location.001-e1718657514350.jpeg" link="#"] Location of the OOI RCA cables off the Oregon coast showing in red the portion of the South Cable extending from the shore station in Pacific City to the first repeater that was successfully interrogated during the experiment. Credit: W. Wilcock, University of Washington.[/media-caption]
In May 2024, a team of scientists led by Dr. Brad Lipovsky at the University of Washington conducted a test of multiplexed distributed acoustic sensing (DAS) along the more southerly of two submarine cables of the OOI Regional Cabled Array (RCA) extending off Pacific City, Oregon. DAS uses backscattered light to measure fiber strain with spatial resolution of meters and at frequencies typically recorded by seismometers. Whereas previous DAS experiments on the RCA sought to demonstrate the use of DAS on OOI RCA infrastructure during a time period when the RCA cables were not being used for communication with the RCA instruments, the May 2024 study sought to demonstrate the use of DAS during a time when active communications were taking place on the same optical fiber as the DAS observations.
The 4-day test was conducted from May 6-10 during a scheduled maintenance shutdown of the OOI RCA. The OOI RCA has two cables extending off Pacific City Oregon, the north cable which extends to Axial Seamount and the south cable that loops back onto the continental margin off Newport to the south of Pacific City. Each cable has two optical fibers, the transmit and receive fibers for observatory data. The multiplexed DAS observations extended to the first optical repeater on the South cable which is located at about 95 km offshore.
The experiment used an Alcatel Subsea Networks (ASN) optoDAS interrogator. From 2024-05-06 15:57:34 to 2024-05-10 14:50:35 UTC, we acquired continuous DAS data on the South cable of the OOI RCA (Figure 1). At 15:00 UTC on May 6 the network was disconnected and we connected the interrogator to the fiber at the outermost module of the submarine terminal system using an in-line C/L filter. Our team tested DAS acquisition parameters for approximately one hour before continuous recording commenced. Around 19:00 UTC on May 7, the network was reconnected to the RCA South cable, through the C/L filter, and the OOI team resumed data telemetry while DAS data acquisition continued. Shortly before 15:00 UTC on May 10, DAS data acquisition was stopped. The C/L filter was then removed, which required briefly disconnecting and reconnecting the RCA South fiber.
The data from the experiment are available by FTP. A description of the dataset is provided via Github repository, including a jupyter notebook that shows how to use the data.. Users may also sign up for a mailing list that will be used to share occasional updates. Note that the total volume of data is about 4 TB. Investigators may contact Mitch Elend to arrange to send hard disk drives which will be returned with the requested data.
Read MoreRCA Technical Wizards: The Saga of Repairing an RCA Primary Node
Chuck McGuire and Larry Nielson were able to take a cabled power and communications substation that sat inoperable on the dark, cold seafloor of the Pacific for two years and make it work again. The odds were against them. There’s usually less than a 50-50 chance to make a complex technology operational again after this length of inactivity – inactivity compounded by the extreme pressure, cold temperature, and biofouling that occurs on the bottom of the Pacific Ocean. But these two engineering geniuses succeeded in repairing, rebuilding, and ultimately reconnecting Primary Node 1B (PN1B), which provides power to the Southern Hydrate Ridge portion of OOI’s Regional Cabled Array (RCA).
[embed]https://vimeo.com/user110037220/pn1b[/embed]It was an arduous, complicated journey, fraught with delays outside of the engineers’ control. The recovery, repair, and reinstallation took two years to complete. We share some of the highlights below for they illustrate the many challenges of operating seafloor cable infrastructure on an active tectonic plate in the Pacific Ocean.
Chuck McGuire, a senior principal engineer, is the chief engineer for the RCA and principal investigator for the engineering portion of the RCA, which is conducted at the University of Washington’s Applied Physics Laboratory (APL). Larry Nielson is a field engineer three at APL. They have been working on the RCA project since its inception in 2009 (Construction and deployment began in 2013).
When PN1B failed in 2020, the duo was stymied at first. They had to call in a variety of people with different skills to recover, rebuild, and redeploy it.
“Restoration of the PN1B is really a testament to the engineering that went into these nodes, which are good and work well under very harsh conditions, “explained McGuire. The Primary Nodes were designed and built by L3 Maripro with oversight by the APL Engineering team.
A Cable Fault Causes Power Loss
The saga began during RCA’s annual maintenance expedition in summer 2020. Everything was smooth sailing until suddenly the science team aboard the R/V Thomas G. Thompson learned of a cable fault on the southern cable line, which had shorted out. McGuire and team instantly went into troubleshooting mode and determined that the problem was in PN1B, which powers the Southern Hydrate Ridge section of the 900 kilometers of cable that comprise the RCA.
[media-caption path="https://oceanobservatories.org/wp-content/uploads/2023/03/202108-Recovery-of-Failed-PN1B-scaled.jpg" link="#"]Recovery of failed PN1B. Credit: NSF/OOI/UW.[/media-caption]Luckily, they were able to divert the ship, the R/V Thomas G. Thompson, to the PN1B location to investigate. McGuire was on shore at the Shore Station in Oregon where he could troubleshoot the power system. McGuire worked with a team onboard to use the Remotely Operated Vehicle (ROV) Jason, operated by the National Deep Submergence Laboratory of the Woods Hole Oceanographic Institution, to unplug and plug back in the science interface assembly (SIA) that provides power and communication to secondary infrastructure on the array (e.g. extension cables, junction boxes, instruments, and moorings). The SIA was attached to and powered by PN1B. The SIA wasn’t the cause of the short, so the next step was a visual inspection of the cable with the ROV, tracing the cables back from the node to see if there was any external aggression or damage to that cable. None was seen. The next step was to determine where the power was lost. By controlling the power from the Shore Station and sending “tones” (an oscillating frequency) down the cable, they were able to isolate the affected area. They determined that the PN1B was no longer transmitting any power to the southern cable line running east of the node.
McGuire and Nielson set to figuring out how to replace the damaged node with a spare. A specialized cable ship would be required. A request for proposal was released and the cable ship IT Integrity was secured.
With a cable ship lined up, the engineering team set about reconfiguring the Primary Node and a replacement SIA, which would be plugged into PN1B when both were back on the seafloor. The assembly was complicated and required reconfiguration of all the optics in the SIA’s interior. “Larry was able to get the SIA unassembled and rigged up in a way that made it possible for me to go in and remove and replace the components that needed to be replaced,” explained McGuire. “All of this was unexpected work, which took time, and there was much aggravation. But Larry had some amazing solutions to some interesting rigging problems.”
The two engineers had to rely on their experience to tear down the SIA and then reconstruct it. Their challenge included having to create specialized tools to get into extremely tight spaces. McGuire likened the work to repairing a car engine, where one slowly takes things off, working through the engine until finding the problem. The difference between the Primary Node and a car, however, was that spare parts to fix any issues found weren’t readily available and would have to be manufactured if needed. Here, again, the skill, experience, and tenacity of this duo were called into play. After taking the node apart and putting it back together again, they are now experts.
Their work was driven by a tight schedule needed to get the node and SIA ready for the next RCA recovery and deployment cruise in July 2021. The plan was to have the cable ship work simultaneously with the ROV Jason that was aboard the Thompson. During the 2021 expedition, Jason successfully unplugged and recovered the SIA. The cable ship Integrity then dropped a recovery line next to the node and Jason hooked the recovery line to the node. The cable ship recovered the node, returned it to the dock in Newport, where it picked up the replacement node. All seemed to be going well and on schedule.
While the transit and offloading were taking place, the team had tied the cables to a surface buoy to keep them accessible for reconnection to the new node. When back at the buoy, engineers on the Integrity began the process of jointing—essentially splicing—the tied-off cables and attaching them to new cables connected to the replacement node. When all was connected, the next step was to use a cable winch to lower the cables down to the bottom. Unfortunately, during deployment of the replacement PN1B, the system was damaged which resulted in a shunt fault. Tests indicated the damage was most likely in or very close to the cable termination assembly (CTA) making a repair with the node, at that time, not possible. The replacement PN1B was recovered to deck.
Rather than admit defeat, McGuire came up with the idea to bypass the replacement node and connect the cable downslope from the west to the eastern cable together to provide power and communication to Primary Nodes PN1C and PN1D. McGuire figured that if optical power was too high, communications would fail since PN1B would not be in the system to attenuate the signal. After a few quick calculations, McGuire determined what the attenuation needed to be in the line so that the power wasn’t too high to prevent communication down the cable. The required attenuators were driven down to Newport while the ship headed to port to retrieve the critical parts. The cables were then jointed with the attenuators in the optical circuit, restoring about 65 percent of the power and communications lost to the cable system initially and allowing RCA instruments and moorings at the Oregon Offshore and Oregon Shelf sites to become operational again. The maneuver was innovative and an engineering feat.
Upon returning to the lab, the team faced other challenges. They had to reverse engineer a CTA. Because many of the parts could not be seen without destroying other critical parts, a CT scanner was used to scan its many parts, and point clouds (discrete set of data points in space) were developed. These cloud points were then fed into Solidworks, a software program, to create models of each part that needed to be machined or refurbished.
“CTAs are a very complex component,“ explained McGuire. “They terminate a cable into an object that must retain its watertight integrity at over 3000 meters water depth, while withstanding 35,000 pounds of pulling force and protecting the optical fibers in the cable. Also, there’s not a rock solid on-land way to test its water tight integrity once it’s been built with the cable, ready for deployment.”
In getting ready for the next deployment attempt, the engineering duo encountered other obstacles from COVID-related delivery delays to manufacturers who no longer manufactured needed components. They persevered. The team tore down the replacement node a second time and rebuilt it, with the same deliberate process given the first replacement node. Once completed, they took the replacement node through a complete battery of tests, making sure that it was capable of being powered up, could communicate with the SIA, and provide power to the cabled system. They then shipped the node to Portland to be loaded on a larger cable ship, the C.S. IT Intrepid.
In June 2022, the replacement node was again ready for deployment. McGuire boarded the Intrepid with field engineer Paul Aguilar to oversee deployment. After two days of bad weather, the ship finally was on site. The deployment went without incident except that the node landed 400 meters short of where it was to connect to the cable. To reposition the node, PN1B would have to be lifted back on deck and four kilometers of cable would have to be “peeled” off the seafloor. This would greatly increase the risk to PN1B and the cable, so the team came up with the idea of essentially creating an extension cord to make the cables meet.
“The node was working, the whole system was working except we couldn’t plug anything into it yet, because it was too far away from where it needed to be,” said McGuire. “That was in the middle of June. We had until the end of July, (for the 2022 RCA recovery and deployment expedition) to acquire the parts, build an extension cable and come up with a plan to connect the two cable segments. “
As they do, McGuire and Nielson came up with a plan. By the July departure date, McGuire was aboard the Thompson to oversee the connection. Nielson was onshore to support logistics and attend to any contingent plans that might be needed. The deployment included coming up with a new rigging configuration that required hanging 500 meters of cable off the bottom of the Remotely Operated Platform for Ocean Sciences (ROPOS), a 40 hp Science/Work Class ROV operated by the Canadian Scientific Submersible Facility, using Yale grips every 100 meters or so to allow for proper positioning once the cable was on the sea floor. Using ROPOS, the team guided the end of the cable lit with a transponder and put the end right next to the Primary Node. They then “walked” the ship towards where the cable had to be plugged into the node, essentially laying out a long string in the water to the correct spot.
“And it worked on the first shot, which still amazes me today,” concluded McGuire. “I cried and went to bed.”
Read More
New OOI Coastal Surface Mooring Design
The OOI Coastal Surface Moorings (CSMs) showcase a variety of innovative mooring technologies. Although the design concepts were initially developed as elements of other mooring systems, they were brought together for the first time on the OOI CSM. A recent paper by Peters et al. (2022) describes three areas where new design concepts were particularly impactful: (1) components at the interface between the surface buoy and mooring riser, (2) mooring riser components, and (3) an integrated seafloor anchor and instrument frame. These components work together to provide mechanical integrity for the mooring as well as mounting points for instrumentation and a reliable electrical pathway for the transmission of power from the surface to the seafloor and data from the seafloor to the buoy (Figure above).
Components comprising the buoy-to-riser interface include a universal joint, an electro-mechanical (EM) chain, and a Near Surface Instrument Frame (NSIF). The universal joint, at the buoy base, reduces the translation of buoy pitch and roll motion into bending moments at the top of the mooring riser. The EM chain, a conventional chain wrapped with helically-wound conductors and encapsulated in urethane, provides a flexible strength member between the buoy and the NSIF. The NSIF provides a mounting point for instruments and a mechanical transition from the EM chain to the EM cable.
Components along the mooring riser include the EM cable, EM stretch hoses, and distributed buoyancy elements between stretch hoses. The EM cable employs a wire rope strength member mechanically terminated with a swaged fitting. A molded urethane strain relief boot at the upper end of the cable interfaces with the NSIF bellmouth. The lower termination assembly provides strain relief and a cavity for integration of underwater connectors. The EM stretch hoses were initially developed in the 1990s by WHOI engineer Walter Paul. A novel, multi-layer construction technique was developed for OOI to enable a 24-conductor stretch hose. Hose lengths from 9 to 30 m are employed on OOI moorings. Each hose has a breaking strength of over 10,000 lb and stretches to over twice its original length to provide variable mooring scope and reduction of peak dynamic loads.
At the buoy base is an integrated anchor and instrument frame assembly called the Mult-Function Node (MFN). The MFN frame is a buoyant structure made of air-filled aluminum pipe, configured to allow the mounting of instruments, data loggers and batteries. The MFN is weighted to the seafloor with an anchor assembly that sits in the center of the frame, connected with dual acoustic releases. The anchor assembly consists of a flat-plate anchor below a foam buoyancy element that contains an internal spool with several hundred meters of synthetic line. The buoyancy element is connected to the anchor with dual acoustic releases. This system allows for mooring and anchor recovery in three stages: First, the MFN is separated from the anchor and the mooring riser is recovered. Next, the buoyancy element is released from the anchor allowing it to rise to the surface while offspooling line. Finally, the anchor is hauled using the synthetic line.
This unique combination of design elements creates a mooring system capable of housing and powering complex instrument systems, transmitting data in near real-time, and contributing to the long-term reliability of the OOI Coastal Surface Moorings in the challenging environment of the continental shelf.
Peters, D.B, J.N. Kemp and A.J. Plueddemann (2022). Coastal Surface Mooring Developments for the Ocean Observatories Initiative (OOI). Marine Technol. Soc. J., 56(6), 70-74. doi.org/10.4031/MTSJ.56.6.2.
Read MoreImproving Reliability and Availability of Dissolved Oxygen Glider Data
OOI’s Coastal Endurance Array Team is making great strides in ensuring the accuracy of glider dissolved oxygen measurements and making these data readily available to researchers. The team has compared glider oxygen data with independent transects and climatologies compiled by west coast colleagues. They are increasing the number of in situ comparisons of glider oxygen data with bottle samples taken in proximity to the gliders. They have built a benchtop system to do two-point calibrations for oxygen sensors to help ensure their accuracy when the gliders are in the water. And, working with OOI colleagues at WHOI, the team is helping to design and test improved sensor mounts to perform in situ air calibrations of glider oxygen measurements.
“A couple of years ago, Endurance Glider Lead Stuart Pearce developed code to put all of the oxygen data collected by our glider fleet into the DAC, the national central database for glider data,” said Ed Dever, Principal Investigator of the Coastal Endurance Array and Professor at Oregon State University. “Prior to doing this, Stuart examined the data and metadata for any gross errors and issues. That was the first of many steps we have taken to ensure OOI oxygen data are reliable and available so they can be trusted and used by researchers. Our effort is paying off. The glider data available on the DAC are identical to that now available on OOI’s Data Explorer. Endurance glider lines off Newport, OR and Grays Harbor, WA will be the backbone for the northern Californian Current part of the nascent Boundary Ocean Observing Network. The OOI glider lines map seasonal development of hypoxic areas off Oregon and Washington from the coast to the edge of the continental shelf.”
[media-caption path="/wp-content/uploads/2022/11/glider.png" link="#"]More than 137 glider missions have occurred along the Newport and Grays Harbor lines since 2014. Credit: Coastal Endurance Array at OSU.[/media-caption]
Collaborations
Enough oxygen data has been collected and shared in the northeast Pacific that is now possible to make high resolution regional climatologies of temperature, salinity and oxygen. Risien et al. (2022) compiled ~20 years of data taken by Jennifer Fisher (NOAA) and many others, to develop analysis ready transects of water property data off Newport, Oregon. These datasets include both climatologies and individual interpolated, quality-controlled, transect data. The Endurance Team compared glider dissolved oxygen with shipboard CTD samples collected by Fisher along the Newport transect in July 2021. Oxygen measurements from the gliders and CTDs samples compared well and provided confidence that glider and shipboard transects can map hypoxic conditions at comparable resolution and accuracy. Added Dever, “We were able to do a side-by-side comparison of the temperature, salinity, and oxygen data collected by the gliders over our Newport hydrographic line with the CTD data sampled by the Fisher’s team using the R/V Elakha. The comparison was quite good and served to validate the quality of the glider data that are an integral part of the Endurance Array.”
Engineering Solutions
A couple years ago, team member Jonathan Whitefield worked with Scripps Institution for Oceanography to build an oxygen Winkler titration rig so the team can titrate its own oxygen samples. The system can be used both in the lab and onboard to validate glider and moored oxygen data. The titration rig has been used to increase the number bottle samples taken during glider deployments and recoveries and to reduce the time between sample acquisition and analysis.
Building on this, Whitefield and others recently assembled in-house two-point calibration baths for pre- and post deployment of oxygen data collected by gliders and moorings. Calibrations are performed at anoxic and near-saturated conditions. The two-point calibration system is based on a similar one used at Scripps Institution for Oceanography (López-García, P., et al., 2022). A sodium sulfite solution is injected into bottle samples to get the low endpoint oxygen calibration point. The high endpoint oxygen calibration point is achieved using a stirred bath at 10°C to get to near saturation. The high endpoint oxygen is measured with replicate Winkler titrations. The calibration baths better ensure accuracy by making it possible to perform routine in-house checks of vendor calibrations on glider and moored sensors as well as identify calibration drift after deployment.
What’s ahead
Oxygen data are reviewed weekly by operators and annotated. Real-time automated quality control using QARTOD tests is in development. In-situ air calibration tests of oxygen optodes are planned for spring 2023. At the suggestion of outside investigators, OOI’s Coastal and Global Scale Node team at Woods Hole Oceanographic Institution modified Slocum glider optode mounts to allow in-situ air calibrations of dissolved oxygen on the Irminger Sea and Pioneer Arrays. The mount, however, affected the flow around gliders with radome fins and caused navigation problems. Modeling done at WHOI showed these problems may be due to vortices of the optode on the starboard side of the fin. The Endurance gliders will test a new mount placement during its upcoming bi-annual operations and maintenance cruise in March of 2023.
“We’re part of the community of practice for glider oxygen calibration and validation,” said Dever. “We engage with colleagues, adopt standard practices and widely share data. This community of users will help all of us get a better handle on ongoing changes in our ocean regions.”
References
Risien et al. (2022) Spatially gridded cross-shelf hydrographic sections and monthly climatologies from shipboard survey data collected along the Newport Hydrographic Line, 1997–2021, https://doi.org/10.1016/j.dib.2022.107922
López-García, et al. (2022) Ocean Gliders Oxygen SOP, Version 1.0.0. Ocean Gliders, 55pp. DOI: http://dx.doi.org/10.25607/OBP-1756. (GitHub Repository, Ocean Gliders Oxygen SOP.
Read More
OOI Launches QARTOD
WHAT IS QARTOD?
As part of the ongoing OOI effort to improve data quality, OOI is implementing the Quality Assurance of Real-Time Oceanographic Data (QARTOD) on an instrument-by-instrument basis. Led by the United States Integrated Ocean Observing System (U.S. IOOS), the QARTOD effort draws on the broad oceanographic observing community to provide manuals for different instrument classes (e.g. salinity, pH, or waves), which outline best practices and identify tests for evaluating data quality. A common code-base is available on GitHub and actively maintained by IOOS partner Axiom Data Science.
OOI has committed to implementing available QARTOD tests where appropriate. QARTOD is well-documented and actively maintained with an engaged user-base across multiple data collection and repository programs. It has a publicly available code-base with standardized tests and flag definitions that result in simplified, easy-to-interpret results. However, some instruments deployed by OOI, such as seismic sensors, hydrophones, and multispectral sensors, are not well-suited towards QARTOD. For instruments for which there is no existing QARTOD manual, such as seawater pCO2, OOI is implementing “QARTOD-like” quality control (QC).
HOW ARE QARTOD RESULTS COMMUNICATED?
QARTOD utilizes a standardized data-flagging scheme, where each data point for an evaluated variable (e.g. salinity) receives one of the following flags: 1 if the data point passed the test and is considered good; 2 if a test was not evaluated; 3 if the data point is either suspect or interesting/unusual; 4 if the data point fails the test; 9 if the data point is missing. Importantly, QARTOD only Flags data, it does not remove data. In fact, OOI is committed to delivering all available data, whether good or bad; the goal is to provide further information on the possible quality of the data. Furthermore, a flag of 3 does not necessarily mean a data point is bad – it could also mean something interesting or unusual occurred that resulted in the given data point being outside of the expected test threshold.
HOW IS QARTOD IMPLEMENTED BY OOI?
OOI is prioritizing implementation of QARTOD on instruments and variables which are shared across arrays and with broad or high scientific interest, such as CTDs, seawater pH and pCO2, dissolved oxygen, and chlorophyll/fluorescence. OOI is currently implementing the gross range and climatology tests (see this article for a more detailed description of the specific implementation). Note that the climatology test is a site-specific seasonally varying range test and not a World Ocean Atlas-like climatology. These two tests, in addition to other tests under development, utilize thresholds and ranges which are calculated from existing OOI datasets. The code used to calculate the thresholds is publicly available at the oceanobservatories ooi-data-explorations github repository and resulting threshold tables are available at the oceanobservatories qc-lookup github repository. The tests executed and results are added to the datasets as variables named _qartod_results and _qartod_executed, with the relevant tested data variable name prepended (e.g. practical_salinity would be practical_salinity_qartod_results and practical_salinity_qartod_executed). The _qartod_executed variable is a list of the individual results of each of the tests applied stored as a string. The tests applied and the order in which they were applied are stored in the variable metadata attributes. The _qartod_results provide a summary result of all the tests applied.
WHAT IS THE CURRENT OOI QARTOD STATUS?
The IOOS QARTOD manuals define the tests for each instrument class as “Required,” “Recommended,” and “Suggested”. The OOI priority will be the “Required” tests for each instrument class in use by OOI. “Recommended” and “Suggested” test development will be reviewed, with the support of subject matter experts, in the future. The IOOS QARTOD manuals can be found at Quality Assurance/Quality Control of Real Time Oceanographic Data – The U.S. Integrated Ocean Observing System (IOOS).
Current tests under development are gross range (GRT) and climatology (CT). The gap test (GT) will follow next. The syntax and location tests are considered operational checks and are handled within OOI operations and management systems. QARTOD test data will be available in specific locations where OOI supplies data, starting with M2M, and then propagated to THREDDS and Data Explorer. The current status of QARTOD test development and availability is shown in Table 1.
[media-caption path="/wp-content/uploads/2022/10/QARTOD-Table-1.png" link="#"]Table 1: Current Status of Development & Availability[/media-caption]
[media-caption path="/wp-content/uploads/2022/10/QARTOD-Figure-1.png" link="#"]Figure 1: QARTOD “Required” Test Development Timeline[/media-caption]
Read MoreCanadian and OOI Gliders Meet in Pacific
In an important collaborative undertaking, the Ocean Observatories Initiative (OOI) Glider 363 and a Fisheries and Oceans Canada (DFO) Glider crossed paths along Line P, a transect line in the northeast Pacific. This modern day “intersection” provides an opportunity for scientists to have co-located science profiles to match up with sensor data, but also an efficient way to extend data about ocean conditions along Line P throughout the year.
Line P consists of 27 stations extending from Vancouver Island to Ocean Weather Station Papa (OWSP), also known as “Station Papa.” OSWP (located at 50°N, 145°W) has one of the oldest oceanic time series records dating from 1949-1981. This 32-year-old record is supplemented by data collected by shipboard measurements along Line P conducted by DFO three times/year. The US National Oceanic and Atmospheric Administration also has a surface mooring at Station Papa, which contributes year-round data to this important record. Beginning in 2014, OOI also enhanced Station Papa with an array of subsurface moorings and glider measurements.
An important intersection
[media-caption path="/wp-content/uploads/2022/09/image012.png" link="#"]The OOI glider left from Newport, OR aboard the RV Zephyr and was deployed on July 3 in open ocean over the Juan de Fuca Ridge. The glider transited along Line P to the Papa Array starting from station P16. A DFO glider was traversing Line P at the same time, providing an opportunity for US and Canadian scientists to have co-located profiles to match up with sensor data. [/media-caption]The DFO glider was deployed in late May returning from OWSP. The OOI glider left from Newport, OR aboard the RV Zephyr and was deployed on July 3 at 46 N 130W in open ocean over the Juan de Fuca Ridge. The glider transited along Line P to OOI’s Global Station Papa Array starting from station P16, which is in international waters just outside the Canadian EEZ. A DFO glider was traversing Line P at the same time, providing an opportunity for US and Canadian scientists to have co-located profiles to match up with sensor data.
At the point of the cross-over the OOI glider had been at sea for about 40 days. Both OOI’s and DFO’s glider have very similar sensors onboard that measure temperature, salinity, pressure, oxygen, optical backscatter, chlorophyll, and colored dissolved organic matter. These measurements when compared to historical data provide insight into existing and possibly changing conditions in the water column.
“At a very basic level these deep-ocean rendezvous provide us with an opportunity to compare the sensor data mid-deployment, instead of just at the start or end of their respective deployments. This can help us look for any trends or offsets that might indicate sensor issues – such as aging, fouling, and other issues that may impede performance. This information helps people understand and be able to use data from these gliders,” explained Peter J. Brickley, OOI’s Glider Lead. “The other outcome is that our joint glider data can contribute extra sampling along Line P. While there are several cruises along this line every year, those efforts are spaced far apart in time (sometimes several months). Autonomous gliders can fill some of the gaps, are relatively inexpensive to operate, and can help better delineate conditions, including changing anomalies as they occur.”
Another contributing factor to making this initial glider cross-over a useful test case is that a scheduled DFO Line P cruise on the Canadian Coast Guard Ship John P. Tully was happening concurrently along Line P. The team aboard the Tully started sampling in early August and are scheduled to complete sampling by the month’s end. The ship collected some data in the vicinity of both gliders, offering another opportunity to compare and contrast data.
[media-caption path="/wp-content/uploads/2022/09/zephyr2.png" link="#"]The OOI glider deployed from the R/V Zephyr heading north to reach Line P in time to cross-over with DFO’s glider to share and contrast data collected. Credit: R/V Zephyr ©WHOI. [/media-caption]While the glider cross-over is an important first, it is emblematic of the ongoing cooperative effort between the Canadian DFO, NOAA, and OOI teams sampling in this important region. Communications occur regularly between OOI team members and the Chief Scientist conducting DFO shipboard sampling, as well as between OOI and NOAA personnel.
Added Brickley, “This recent excursion along Line P was planned, but also a serendipitous opportunity that could be leveraged quickly. Once we all have the chance to assess the data provided, we’ll be in a better position to explore making this a more regular occurrence. If it turns out that our sampling schemes are easily aligned, that could be another step to help advance understanding of ocean processes from coastal, eutrophic waters into the heart of the high nitrate, low chlorophyll area of the NE Pacific.”
Read More
Measurements Below the Surface
Strong winds and large waves in remote ocean locations don’t deter the Ocean Observatories Initiative (OOI) from collecting measurements in spite of such extreme conditions. By moving the moorings below the surface, the OOI is able to secure critically important observations at sites such as the Global Station Papa Array in the Gulf of Alaska, and the Global Irminger Sea Array, south of Greenland. These subsurface moorings avoid the wind and survive the waves, making it possible to collect data from remote ocean regions year-round, providing insights into these important hard-to-reach regions.
Instrumentation on the surface mooring in the Irminger Sea, however, has nowhere to hide and the measurements they provide are also often crucial for investigations, such as net heat flux estimates. Providing continuous information about wind and waves remains one of the most challenging aspects of OOI’s buoy deployments in the Irminger Sea. Fortunately, with each deployment, OOI is improving the survivability of the surface mooring so they continue to add to the valuable data collected in the region by their subsurface counterparts.
[media-caption path="/wp-content/uploads/2022/07/FLMB-9_DSC_0934.jpg" link="#"]The top sphere of a Flanking Mooring being deployed through the R/V Neil Armstrong’s A-Frame. Credit: Sawyer Newman©WHOI.[/media-caption]Below the surface in the Irminger Sea
A team of 15 OOI scientists and engineers spent the month of July in the Irminger Sea aboard the R/V Neil Armstrong, recovering and deploying three subsurface moorings there, along with other array components. The Irminger Sea is one of the windiest places in the global ocean and one of few places on Earth with deep-water formation that feeds the large-scale thermohaline circulation. Taking measurements in this area is critical to better understanding changes occurring in the ocean.
OOI’s Irminger Sea Array also provides data to an international sampling effort called OSNAP (Overturning in the Subpolar North Atlantic) that runs across the Labrador Sea (south of Greenland), to the Irminger and Iceland Basins, to the Rockall Trough, west of Wales. The OOI subsurface Flanking Moorings form a part of the OSNAP cross-basin mooring line with additional instruments in the lower water column. During this current expedition, the Irminger Team will be recovering and deploying OSNAP instruments that are included as part of the OOI Flanking moorings, in addition to turning several OSNAP moorings as well.
[media-caption path="/wp-content/uploads/2022/07/FLMB-9_DSC_0941.jpg" link="#"]The Flanking Mooring top float in the water during deployment. The sensors mounted in the sphere will measure conductivity, temperature, fluorescence, dissolved oxygen and pH at 30 m depth. Credit: Sawyer Newman©WHOI.[/media-caption]The triangular array of moorings in the Irminger Sea provide data that resolve horizontal variability, how much the physical aspects of the water (temperature, density, currents) and its chemical properties (salinity, pH, oxygen content) change over the distance between moorings. The individual moorings resolve vertical variability – the change in properties with depth. Three of these moorings are entirely underwater, with no buoy on the surface. They do have, however, multiple components that are buoyant to keep the moorings upright in the water column.
[media-caption path="/wp-content/uploads/2022/07/FLMB-9_DSC_0984.jpg" link="#"]The mid-water sphere holds an ADCP instrument which will measure a profile of water currents from 500 m depth to the sea surface. Photo Credit: Sawyer Newman©WHOI.[/media-caption]Each subsurface mooring has a top sphere at 30 m depth, a mid-water sphere at 500 m depth, and back-up buoyancy at the bottom to ensure that the mooring can be recovered if any of the other buoyant components fail. Instruments are mounted to the mooring wire to make measurements throughout the water column.
[media-caption path="/wp-content/uploads/2022/07/FLMB-9_IMG_5328.jpg" link="#"]Glass balls in protective “hard hats” provide extra flotation at the bottom of the mooring. Their tennis ball yellow color looks almost fluorescent in the brief (and much enjoyed) sunshine. Photo Credit: Sheri N. White©WHOI.[/media-caption] Read MoreRemotely Fixing and Preventing Mooring Issues
Alex Franks’s job is a big one. He is charged with fixing various issues that occur on OOI moorings, while they are hundreds and sometimes even thousands of miles away in the ocean. As an Engineer II at Woods Hole Oceanographic Institution (WHOI), Franks is intimately familiar with the mooring system controller software, which allows him to troubleshoot and fix instrumentation problems on OOI moorings, regardless of their location.
Franks has been working with electronics for over a decade and solving OOI mooring-related challenges since 2015. Many examples exist of his innovative solutions. In 2020, for example, the satellite Internet service that was being used to send data from OOI moorings to WHOI servers was no longer a viable solution. The WHOI team faced the task of either finding a replacement system, or working with the then-current system. One easily implementable solution was to move to transmitting data through OOI’s Iridium radio antennas full time. There were downsides to this solution, however. It would allow no margin of error, would consume more power, and still not be able to send data from all the instruments.
Franks figured out a better solution that would both keep costs manageable and continue to meet timely data transmission goals by modifying the Iridium file transfer portion of the mooring software to accommodate a new data transfer scheme. The new scheme used a feature of the computer program rsync, a fast and versatile file copying tool, called “diff”. Instead of using rsync to communicate with shore servers and determine the “delta” or change between the new instrument data on the mooring and the instrument data files on the WHOI server, he used one of the mooring’s onboard computers as an intermediary server to generate “diff” files against (delineating old from new data). These files were then generated and stored, and sent over the Iridium connection. Using this new configuration, Franks succeeded in sending the entire dataset of all instruments on the mooring, except one that was sent at a reduced sample rate. While transmission times can vary with weather conditions, this newly configured system sends data to the server every 20 minutes every other hour, reducing transmission times from 1440 minutes per/day to about 240 minutes per day.
[media-caption path="/wp-content/uploads/2022/04/DSC_0639-copy.jpg" link="#"]Waves in the North Atlantic can get pretty large, which makes it hard to conduct research at sea, especially in winter. The waves and wind in the Irminger Sea also create challenges for ocean observing equipment in the water there year-round. Credit: ©WHOI.[/media-caption]
Franks also found ways to remotely manage mooring issues caused by weather and sea state by modifying software that controls wind turbines. Wind turbines play a critical role on OOI moorings, providing power to recharge the main system batteries. At the Irminger Sea Array, where the sun is absent for months at a time (the moorings also utilize solar panels), these wind turbines are critical. Prior to Franks’ software fix, human input was required to disable the turbines to prevent them from spinning while wave heights were too great. Franks modified the software used to control the spinning of the turbines to read environmental data from the buoy itself and make automated decisions in real-time that previously had to be done manually. The system now changes its configuration based on a variety of sensor inputs, which make for more immediate decisions to ensure the continued safe operations of the turbines. The software modifications not only help mitigate heavy sea damage to the turbines but saves power, as well. The software detects when the air temperature is above freezing and turns off the precipitation sensor heaters, conserving energy when possible. The software also has fail-safes in place for high or low voltage and to determine hydrogen concentration levels inside the electronics. An illustration of this software configuration is provided below.
[media-caption path="/wp-content/uploads/2022/04/Mooring-system-software-upgrade.png" link="#"] This new software configuration detects when the air temperature is above freezing and turns off the precipitation sensor heaters, and has fail-safes in place for high or low voltage and for hydrogen concentration levels inside the electronics .Credit: ©WHOI.[/media-caption]
Franks has also developed software improvements to the power system controller inside the OOI surface moorings. His work ran the gamut from disabling operational bugs in the system to reducing power consumption to fixing software errors to increase reliability. During a year-long deployment in the Irminger Sea, part of the power system controller board failed. Franks installed a software patch remotely that was able to limit the level of charge coming from wind turbines and wrote a fail-safe feature for the system to disconnect all charging sources if the voltage approached dangerous levels.
The challenges are what keeps Franks enthusiastic about his job, “I just love trying to figure out a solution and it’s particularly rewarding to be able to remotely resolve issues with equipment deployed in the open ocean.”
Read More
Uncovering Changing Life in the Water Column
Oregon State University Assistant Professor Jennifer Fehrenbacher needed a ship to carry out her National Science Foundation-funded research investigating the lives of foraminifera (single-celled organisms about the size of a grain of sand and smaller) in the northern Pacific. Her work, in collaboration with Dr. Claudia Benitez-Nelson at the University of South Carolina (UofSC), involves deploying bottom-moored sediment traps and collecting plankton tows while at sea, giving researchers the opportunity to explore foraminifera that live in lighted surface waters, and how these communities have changed over time in response to the surrounding ecosystem.
Fehrenbacher found her ship. She will be joining forces with the Endurance Array Team aboard the R/V Sikuliaq during its bi-yearly expedition to recover and deploy ocean observing equipment at its array in the northeast pacific off the coast of Oregon. Fehrenbacher and her team of four researchers will join the Endurance Array 16 team in early April for the second of its two-leg expedition. This will be a continuation of her research project that began in September 2021.
Last September, Fehrenbacher’s team put in place two tandem sediment traps that are located close to the OOI Slope base node. One was deployed at about 600 meters water depth, the other slightly above the sea floor. The last sediment trap study in this region was conducted around 30 years ago, and the foraminiferal species have likely changed since then, as have ocean conditions.
[media-caption path="/wp-content/uploads/2022/03/IMG_5185-copy.jpg" link="#"]Fehrenbacher’s team will be retrieving two tandem sediment traps that are located close to the OOI Regional Cabled Array slope base node. Credit: Jennifer Fehrenbacher, OSU.[/media-caption]
The sediment traps have been collecting material in place for the past six months and will be recovered along with the Endurance 16 team’s recovery and deployment work. Once the traps are back onboard, the collecting cups will be taken off, emptied, replaced, and the traps redeployed for another six-month period. The researchers will package the collected materials for analysis at OSU and UofSC.
The team also will be examining live specimens from night-time plankton tows, taking advantage of quiet night-time hours to conduct the plankton tows when the Endurance team is unable to work safely moving large, bulky, and unwieldy equipment in and out of the ocean. “This arrangement is a win-win for everyone,” said Endurance Array Chief Scientist Ed Dever. “It maximizes the use of ship time, while helping to provide data to answer some questions about how ocean conditions are changing.”
Fehrenbacher’s team will be conducting a series of discrete new tows from the surface to about 500 meters. “While I don’t anticipate a ton of critters in the really deep water, net tows haven’t been done extensively in these waters at these depths. The last one was 30 years ago by oceanographer Alan Mix and his graduate student Joseph Ortiz, so this new work will give us insight into how life in the water column may have changed over time, “ said Fehrenbacher.
Fehrenbacher’s team will be conducting experiments with live foraminifera in a portable travel lab they will bring onboard. A number of different experiments are planned:
PhD candidate Kelsey Lane will be collecting foraminifera (shortened version “forams”) to study their genetics and their microbial communities, other species living with forams.
Graduate student Grace Meyer will be striving to measure carbon and oxygen isotopes in individual forams. She will be collecting empty shells from both the water column and sediment trap material and compare what is found in both, providing information about water column processes that could alter shells’ composition.
Postdoctoral researcher Brittany Hupp will be collecting both live forams and empty shells to study the chemistry of different types of forams, looking at their isotopes and trace metals content.
Researcher Eric Tappa, a sediment trap expert from the UofSC, will be deploying and recovering the sediment trap equipment. Tappa has been working with sediment trap moorings for decades and have proven critical in providing longer time-series records of processes occurring in overlying waters.
As lead scientist Fehrenbacher will be participating in these onboard experiments. She also will be growing forams under controlled conditions and watching them to learn how temperature modulates their shells or their behaviors during the day-night cycle, and will continue her work with recovered forams once she returns to her home lab. There she will be measuring the trace element concentrations in foram shells so results can help inform the Paleo record. . She explained, “Foram shells are used basically as proxies for environmental conditions. So when we measure trace elements in their shells, this gives us information about the pH, temperature, nutrient content, and even the salinity of the ocean in the past.” The onboard experiments help scientists under how forams incorporate these elements into their shells when they are alive, which they can use in assessing past records.
[media-caption path="/wp-content/uploads/2022/03/IMG_5196-copy.jpg" link="#"]An anchor weighing ~2500 pounds is deployed to keep a sediment trap in place for six months until it is recovered with a treasure trove of marine life and particulates for investigation. Credit: Jennifer Fehrenbacher, OSU.[/media-caption]
Challenges of studying small living things
Studying single-celled organisms is difficult on dry land, but is compounded by a moving ship in rolling seas. Fehrenbacher’s team will be taking onboard multiple microscopes, including an inverted microscope to see their subjects, water circulators to keep them at constant conditions while they are alive, and a pH meter. High-powered microscopes are critical for the work because forams range in size from smaller than a grain of sand—about 100 microns— to up to about a millimeter.
“We look at forams in a petri dish under the microscope,” explained Fehrenbacher. “This is challenging as the ship moves back and forth so does the water in the petri dish so you’re looking back and forth as the ship moves.” The researchers have come up with the solution of holding the petri dish at an angle so the water and foram stay in one place and helps prevent researchers’ sea sickness.
Fehrenbacher predicts that the amount of material collected on the Endurance Array 16 cruise will keep she and her graduate students busy for at least the next two years and beyond. She added, “There’s really just nothing quite like the type of information you can get from sediment trap studies. While going out to sea for two weeks a year and doing plankton tows are helpful, we only get information about what’s in the water column at the exact moment of collection. But sediment traps provide months’ worth of data at really high resolution that we can compare with other OOI data and get a detailed picture of ocean conditions and how those conditions affect marine life.
Read More
Improving Remote System Response in Increasingly Hostile Oceans
Wind and Waves and Hydrogen, Oh My!
Improving remote system response in increasingly hostile oceans
This article is a continuation of a series about OOI Surface Moorings. In this article, OOI Integration Engineer Alexander Franks discusses the mooring software and details some of the challenges the buoy system controller code has been written to overcome.
Components of the OOI buoys working in concert make up a system that is designed for deployment in some of the most challenging areas of our world’s oceans. These systems collect valuable scientific data and send it back to Wood Hole Oceanographic Institution (WHOI) servers in near real time. Mechanical riser pieces (wire rope, and/or stretch hoses) moor the buoy to the bottom of the ocean. Foam flotation keeps the buoy above water in even the worst 100-year storm, while its masthead supports instrumentation and satellite radios that make possible the continuous relaying of data. The software controlling the system is just as important as the physical aspects that keep the system operating.
The system software has a variety of responsibilities, including setting instrument configurations and logging data, executing power schedules for instruments and parts of the mooring electronics, controlling the telemetry system, interfacing with lower-level systems including the power system controller, and distributing GPS and timing. The telemetry system is a two-way communication path, so the software controls data delivery from the buoy, but also provides operators with the ability to perform remote command and control.
[caption id="attachment_22938" align="alignnone" width="745"]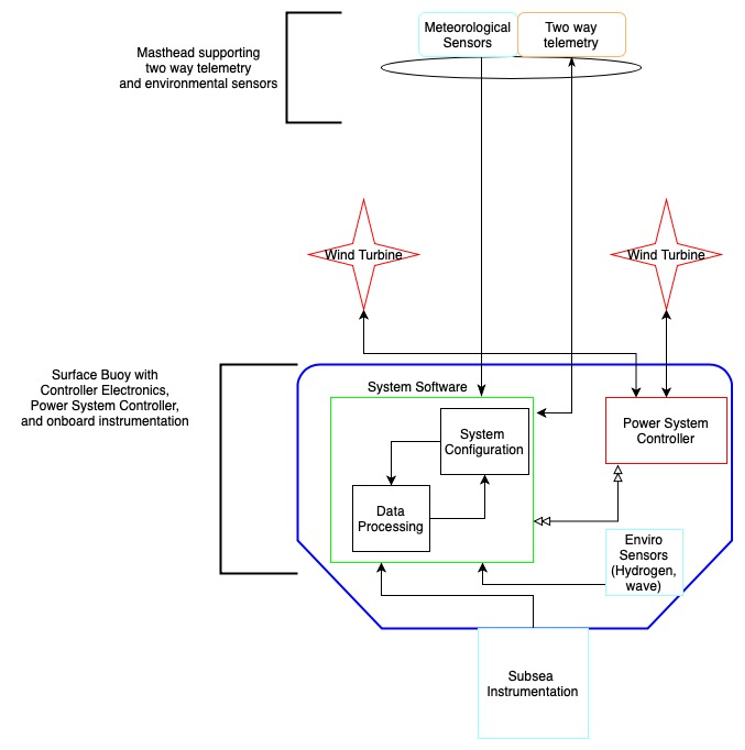
The unforgiving environment and long duration deployments of OOI moorings lead to occasional system issues that require intervention. Huge storms, for example, can build waves so high that they threaten wind turbines on the moorings. At the Irminger Sea Array, ice can accumulate so much as to drastically increase the weight of the masthead, and with subsequent buoy motion, risk dunking the masthead and instruments. Other mooring functions require constant attention. The charging system must be monitored to ensure system voltages stay at safe levels and hydrogen generation within the buoy itself is kept within safe limits. Two-way satellite communication allows operators to handle decision making from shore using the most up-to-date information from the buoy.
“Since starting in 2015 and following multiple mooring builds and deployments, I’ve realized that issues can rapidly arise at any time of the day or night. I started thinking about what the buoys can do for themselves, using the data being collected onboard,” Franks said.
One of the game-changing upgrades implemented by Franks was to read environmental data and make automated buoy safety decisions in real-time that were previously performed by the team manually. For example, previously, the team would need to monitor weather forecasts and decide preemptively whether changes to buoy operations were advisable. With recent software changes, the system can now change its configuration based on a variety of sensor inputs. These variables include system voltage, ambient temperature, hydrogen levels inside the buoy well, wind speed, and buoy motion (for sea state approximation). In addition to the software updates, the engineering team redesigned the power system controller. They added charge control circuits and the ability to stop the wind turbines from spinning. The software and electrical upgrades now provide redundant automated safeguards against overcharging situations, hydrogen generation, and turbine damage, maximizing buoy operability in harsh environments.
[caption id="attachment_22946" align="alignleft" width="650"]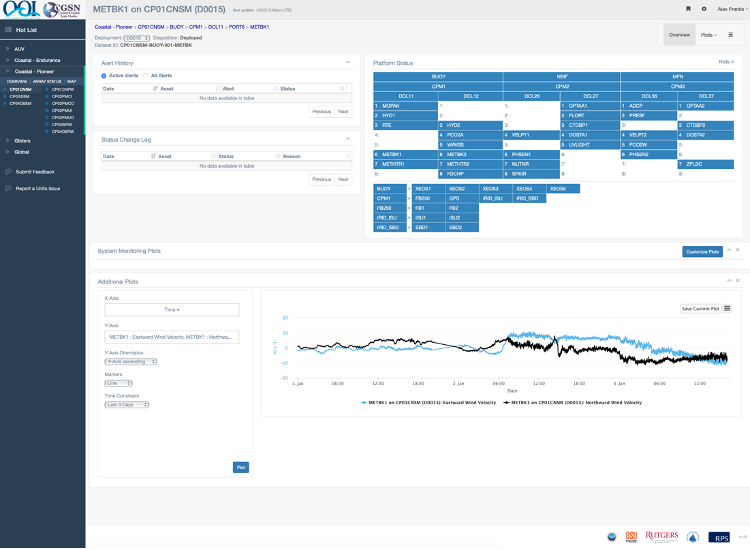
With a largely independent system, operators also needed a way to easily monitor status of the buoys and instrumentation. The software team created a new shoreside dashboard that allows operators to set up custom alerts and alarms based on variables being collected and telemetered by the buoy. While the buoy systems can now operate autonomously, alerts and alarms maintain a human-in-the-loop component to ensure quality control.
As operations and management of the moorings have progressed, the operations team has found opportunities to fine tune how operators and the system handle edge cases of how the system responds to hardware failures and extreme weather. In the past, sometimes conditions changed faster than the data being transmitted back to shore. This new sophisticated software automates some of the buoy’s responses to changing conditions in real time, which helps to ensure their continued operation even under challenging conditions. The decreased response time to environmental and system events using an automated system, coupled with the ability to monitor and interact remotely, has increased the reliability and survivability of OOI moorings.
Read More