Science Highlights
Irminger Sea Carbon Cycle
The high-latitude North Atlantic, a region with high phytoplankton production in the spring and deep convection in the winter, is of particular importance for the global carbon cycle. The vertical transport of carbon from near the surface into the deep ocean, by combination of biological and physical processes, is known as the biological carbon pump. The carbon pump is particularly active in the Irminger Sea, yet the carbon budget, and its seasonal and interannual variability, are poorly known. A study by Yoder et al. (2024) used carbon system data from multiple observational assets (moorings and CTD casts) of the OOI Irminger Sea Array to assess net community production in the mixed layer and the implications for the biological pump in this region.
Data analysis was challenging, because it involved working with multiple instrument types, gappy records, calibration offsets, and other idiosyncrasies. In addition, data from multiple instruments and observing platforms needed to be combined to produce continuous records. The primary sensors utilized were pH and pCO2. These are difficult sensors to work with, to the extent that a community workshop was convened to develop a “users guide” to best practices for analysis (Palevsky et al., 2023). Yoder et al. were able to quality control, cross-calibrate, and merge data from the OOI surface mooring, flanking moorings, gliders and shipboard CTD casts (Fogaren and Palevsky 2023; Palevsky et al. 2023) to create the first multi‐year time series of the inorganic carbon system for the Irminger Sea mixed layer. This remarkable data set, based on instruments with sample rates of 1-2 hours, provides a seven-year record with near-daily resolution (Figure 28).
The time series results (Figure 3) showed that carbon system variables (dissolved inorganic carbon (DIC), total alkalinity (TA), and partial pressure of CO2 (pCO2)) co-vary through the annual cycle, with minimums in late summer at the end of the productive season and maximums in winter. The summer draw-down of pCO2 indicates that biophysical effects, rather than temperature, are the primary drivers of pCO2 variability. The influence of vertical mixing and primary productivity can be clearly seen in DIC and TA. In the subpolar North Atlantic, shoaling of the mixed layer in spring is generally associated with spring phytoplankton blooms, as indicated by increasing chlorophyll (Chl) concentration. Interestingly, it is found that highest integrated rates of DIC removal from the mixed layer via photosynthesis take place prior to mixed layer shoaling.
After a thorough analysis that included mixed layer budgets of DIC and TA, followed by assessment of gas exchange, physical transport, and the hydrologic cycle, the authors conclude that strong biological drawdown is the primary removal mechanism of inorganic carbon from the mixed layer. Furthermore, they point out the importance of interannual variability in both the drivers of and resulting magnitude of surface carbon cycling. This is primarily due to variability in net community production. Acknowledging the challenges taken on by OOI to maintain an array in the Irminger Sea, the authors note that “collecting observational data is both costly and challenging, however if only 1 year of data is collected or multiple years are averaged together, [carbon system dynamics] … will be misrepresented.”
This project shows the potential for OOI data, with appropriate processing and analysis, to provide unique insights into the ocean carbon system. It is notable that the authors made a substantial effort to calibrate and combine data from multiple instruments and moorings, and to take advantage of ancillary data (e.g. gliders, OOI CTD casts, and non-OOI CTD casts) in their processing. Enabling this type of analysis was a goal in the design of the multi-platform OOI Arrays and shipboard validation protocols.
[caption id="attachment_34983" align="alignnone" width="623"]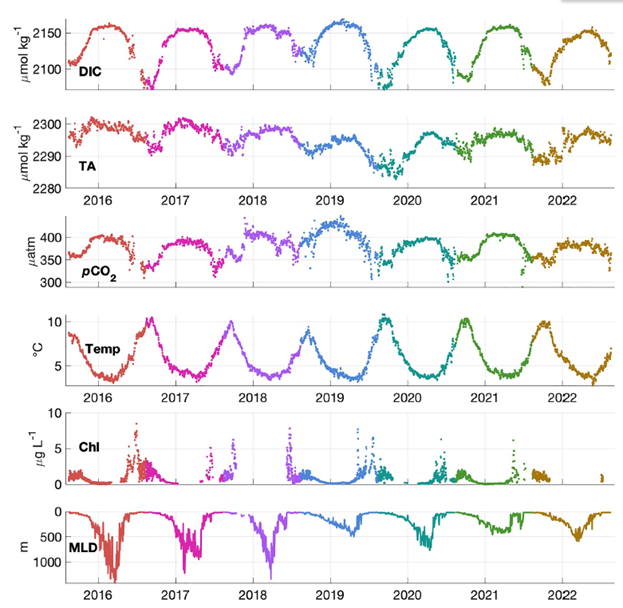
___________________
References:
- Fogaren, K. E., Palevsky, H. I. (2023) Bottle-calibrated dissolved oxygen profiles from yearly turn-around cruises for the Ocean Observations Initiative (OOI) Irminger Sea Array 2014 – 2022. Biological and Chemical Oceanography Data Management Office (BCO-DMO). Version Date 2023-07-19 doi:10.26008/1912/bco-dmo.904721.1
- Palevsky, H.I., S. Clayton and 23 co-authors, (2023).OOI Biogeochemical Sensor Data: Best Practices & User Guide Global Ocean Observing System, 1(1.1), 1–135. https://doi.org/10.25607/OBP-1865.2
- Palevsky, H. I., Fogaren, K. E., Nicholson, D. P., Yoder, M. (2023) Supplementary discrete sample measurements of dissolved oxygen, dissolved inorganic carbon, and total alkalinity from Ocean Observatories Initiative (OOI) cruises to the Irminger Sea Array 2018-2019. Biological and Chemical Oceanography Data Management Office (BCO-DMO). Version Date 2023-07-19 doi:10.26008/1912/bco-dmo.904722.1
- Yoder, M. F., Palevsky, H. I., & Fogaren, K. E. (2024). Net community production and inorganic carbon cycling in the central Irminger Sea. J. Geophys. Res., 129, e2024JC021027. https://doi.org/10.1029/2024JC021027
Impact of Ocean Model Resolution on Temperature Inversions in the Northeast Pacific Ocean
Temperature inversions are a local vertical minimum in temperature located at a shallower depth than a local maximum. In the Northeast Pacific, several water masses and multiple mechanisms for transforming or advecting ocean temperature (cold air events, upwelling, river discharge, cross-shelf eddy transport) create favorable conditions for temperature inversions. Modeling these temperature inversions is challenging. Osborne et al. (2023) analyze observations from 2020 and 2021 to characterize inversions in the Northeast Pacific. The data for these observations come largely from OOI Endurance Array gliders accessed through the GTS database. They compare the observed inversions to model results from the U.S. Navy’s Global Ocean Forecast System version 3.1 (GOFS 3.1) and two instances of the Navy Coastal Ocean Model. Temperature inversions are observed to be present in about 45% of profiles with temperature minimums between 50 – 150 m, temperature maximums between 75 – 175 m, and inversion thickness almost entirely less than 40 m. Modeled temperature inversions are present in only about 5% of model-observations comparisons, with weaker, shallower minimums. This is attributed to two primary causes: coarse model resolution at the inversion depth and the assimilation process which low-pass filters temperature, making inversions weaker. Osborn et al. identify additional work to test the impact of vertical grids on improving model performance.
[caption id="attachment_34977" align="alignnone" width="392"]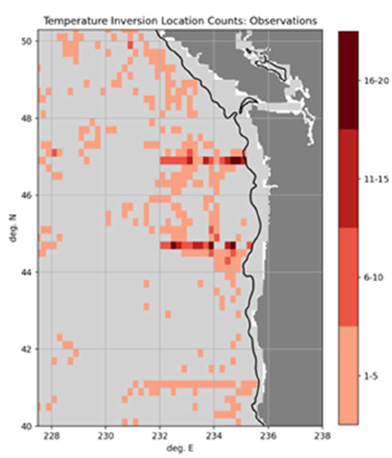
___________________
References:
J. Osborne V, C. M. Amos and G. A. Jacobs, “Impact of Ocean Model Resolution on Temperature Inversions in the Northeast Pacific Ocean,” OCEANS 2023 – MTS/IEEE U.S. Gulf Coast, Biloxi, MS, USA, 2023, pp. 1-8, doi: 10.23919/OCEANS52994. 2023.10337390.
Read MoreNew Hints When Axial Might Erupt: Precursor Events Detected Through Machine Learning
A recent paper by Wang et al., “Volcanic precursor revealed by machine learning offers new eruption forecasting capability” [1] describes the characterization of time-dependent spectral features of earthquakes at Axial Seamount prior to the 2015 using unsupervised machine learning (this method applies algorithms to analyze data without humans in the loop). A major finding from this work is the identification of a distinct burst of mixed-frequency earthquake (MEF) signals that rapidly increased 15 hours prior to the start of the eruption, peaked one hour before lavas reached the seafloor, and earthquakes at Axial Seamount prior to the 2015 using unsupervised machine learning (this method applies algorithms to analyze data without humans in the loop). A major finding from this work is the identification of a distinct burst of mixed-frequency earthquake (MEF) signals that rapidly increased 15 hours prior to the start of the eruption, peaked one hour before lavas reached the seafloor, and migrated along pre-existing faults. The earthquakes are thought to reflect brittle failure driven by magma migration and/or degassing of volatiles. The source mantle beneath Axial Seamount contains extremely high CO2 concentrations leading to high concentrations in the melts [3,4]. MEFs were detected for months prior to the eruption, which could result from volatile release associated with inflation of the sills that feed Axial [5]. Importantly, the identification of these signals may help forecasting of the upcoming Axial eruption and may also be applied to other active volcanoes.
The authors utilized a wealth of geophysical data from the past decade to present an integrated view of Axial (Figure 1) including a subset of earthquake data 4 months prior to the eruption (67,767 out of their >240,000 earthquake catalogue from the RCA seismic array [2]), coupled with bottom pressure from BOTPT instruments at the Central Caldera and Eastern Caldera sites, and 3D modeling.
[caption id="attachment_34973" align="alignnone" width="640"] After Wang et al., 2024 [1] Figures 1 and 4: a) Heatmap of earthquake density at Axial Seamount from Nov 2014 to Dec 2021 [2]. Image highlights mixed‐frequency earthquakes (MFEs – light blue dots) one day before the eruption, the caldera rim (white solid line), the 1.5 km depth contour of the Axial magma chamber (AMC)(dashed white line), eruptive fissures (orange lines), lava flows of the 2015 eruption (yellow lines), the location of the RCA short-period seismometer array (white triangles),broadband seismometer AXCC1 and bottom pressure tilt instruments (MJ03E and MJ03F. Heatmap shows the number of earthquakes in each 25 m × 25 m bins. b) Cartoon summarizing observations. Tidally‐driven earthquakes occur on caldera ring faults, while the MFEs track movement of volatiles and magma prior to the eruption. c) possible mechanisms of the MFEs. ① and ② correspond to crack opening and volatile/magma influx processes.[/caption]___________________
References:
[1] Wang, K., F. Waldhouser, M. Tolstoy, D. Schaff, T. Sawi, W.S.D. Wilcock, and Y.J. Tan (2024) Volcanic precursor revealed by machine learning offers new eruption forecasting capability. Geophyiscal Research Letters, 51 (19) https://doi.org/10.1029/2024GL108631.
[2] Wang, K., F. Waldhouser, D.P. Schaff, M. Tolstoy, W.S.D. Wilcock, and Y.J. Tan (2024) Real-time detection of volcanic unrest and eruption at Axial Seamount using machine learning. Seismological Research Letters, 95, 2651–2662, doi: 10.1785/0220240086.
[3] Helo, C., M.-A. Longpre, N. Shimizu, D.A. Clague and J. Stix. (2011) Explosive eruptions at mid-ocean ridges driven by CO2-rich magmas. Nature Geoscience. 4, 260–263 (2011). https://doi.org/10.1038/ngeo1104.
[4] Dixon, J. E., E. Stolper, E., and J.R. Delaney (1988). Infrared spectroscopic measurements of CO2 and H2O in Juan de Fuca Ridge basaltic glasses. Earth and Planetary Science Letters, 90(1), 87–104. https://doi.org/10.1016/0012‐821x(88)90114‐8.
Read MoreDeep-Ocean Vertical Structure
It is often assumed that, at frequencies below inertial, the vertical structure of horizontal velocity and vertical displacement can be reasonably described by a single dynamical mode, e.g. the lowest order flat-bottom baroclinic mode. This is appealing because it would mean that first-order predictions of deep-ocean velocity structure could be determined from knowledge of density and surface currents. However, there is a relative paucity of full ocean depth data to test this idea. A study by Toole et al. (2023) used full ocean depth data from five sites – four of which are Ocean Observatories Initiative (OOI) arrays (Station Papa, Irminger Sea, Argentine Basin and Southern Ocean) – to address the question “does subinertial ocean variability have a dominant vertical structure?”
Data analysis was challenging, because it involved working with gappy records as well as combining information from multiple instruments on different moorings. As noted by the authors, “no single OOI mooring sampled velocity, temperature and salinity over full depth.” Wire-following profiler data from Hybrid Profiler Moorings were combined with ADCP and fixed-depth CTD data from adjacent moorings. While the authors note that “depth-time contour plots of the velocity data from each OOI site clearly reveal the shortcomings of the datasets” they also recognized that despite the shortcomings, “these observations constitute some of the only full-depth observations of horizontal velocity and vertical displacement from the open ocean.”
It was possible to obtain 2-3 years (non-contiguous in some cases) of near-full ocean depth data from each site. Inertial and tidal variability was removed, and the data were filtered over 100 hr (~4 days). Empirical Orthogonal Function (EOF) decomposition was used to identify an orthogonal basis set that described horizontal velocity and vertical displacement. In addition, dynamical modes were determined for three cases: flat bottom, sloping bottom and rough bottom. Note that computing the dynamical modes requires the vertical density profile, which was taken as the mean over each deployment. Analysis was focused on the lowest modes, which accounted for the majority of the variance.
The results (Figure 32) showed that there is an EOF consistent with a dynamical mode at most sites. However, the appropriate dynamical mode is different for each site – no single dynamical accounted for a dominant fraction of variability across all sites. The authors note that differences in bathymetry, stratification and local forcing complicate the picture, with different dynamical processes dominating at different sites. Prior studies (not full ocean depth) that appear to show a “universal” vertical structure may be misleading
This project shows the potential for OOI data, with appropriate processing and analysis, to provide unique insights into ocean structure and dynamics. The researchers have made the combined vertical profile data available to the community on the Woods Hole Open Access Server. The dataset DOI (https://doi.org/10.26025/1912/66426) is also linked here: https://oceanobservatories.org/community-data-tools/community-datasets/.
[caption id="attachment_34586" align="alignnone" width="624"]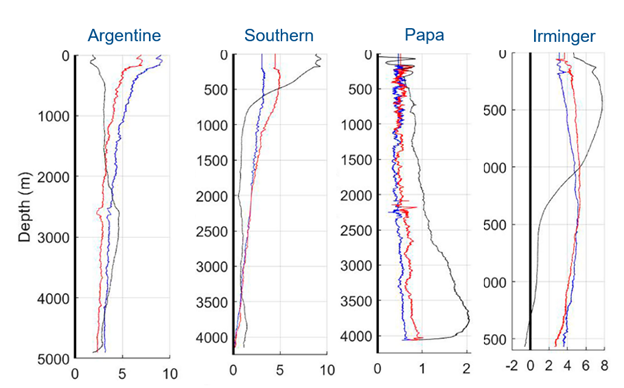
___________________
References:
Toole, J.M, R.C. Musgrave, E.C. Fine, J.M. Steinberg and R.A. Krishfield, 2023. On the Vertical Structure of Deep-Ocean Subinertial Variability, J. Phys. Oceanogr., 53(12), 2913-2932. DOI: 10.1175/JPO-D-23-0011.1.
Read MoreSubsurface Acoustic Ducts in the Northern California Current System
Xu et al.’s analysis of the hydrographic data recorded along the U.S. Pacific Northwest coastline leads to the identification of a secondary subsurface acoustic duct. A numerical simulation based on the sound-speed field determined from OOI Coastal Endurance and APL-UW glider CTD data suggests that the presence of the duct has major impact on sound propagation at a mid-range frequency of 3.5 kHz in the upper ocean (Figure 31). Specifically, the ducting effect is evident in the trapping of sound energy and the consequent reduction in transmission loss within the duct. Glider observations show that the duct is a large-scale phenomenon that extends hundreds of kilometers from the outer continental shelf to regions offshore of the continental slope. The axis of the duct shoals onshore from between 80 and 100 m depth offshore of the continental slope to less than 60 m over the shelf. Analysis of the sound-speed profiles determined from glider CTD data suggests that the prevalence of the duct decreases onshore, from over 40% in regions offshore of the continental slope to less than 5% over the shelf. In addition, analysis of the long-term time series of sound-speed profiles determined from the CTD data recorded over the shelf slope off the Washington Coast suggests that the duct is more prevalent in summer to fall than in winter to spring. Furthermore, examination of concurrent OOI Coastal Endurance Array (Washington Offshore Profiling Mooring) observations of sound speed and flow velocity indicates that the duct observed over the shelf slope is associated with a vertically sheared along-slope velocity profile, characterized by equatorward near-surface flow overlaying poleward subsurface flow.
[caption id="attachment_34581" align="alignnone" width="462"]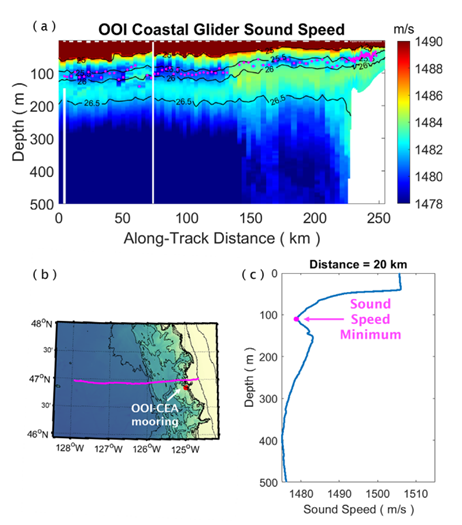
___________________
References:
Guangyu Xu, Ramsey R. Harcourt, Dajun Tang, Brian T. Hefner, Eric I. Thorsos, John B. Mickett; Subsurface acoustic ducts in the Northern California current system. J. Acoust. Soc. Am. 1 March 2024; 155 (3): 1881–1894. https://doi.org/10.1121/10.0024146
Read MoreAxial Seamount: The Phoenix Rises
Regional Cabled Array live data feeds from the bottom pressure tilt, seismic, and temperature-resistivity instruments are capturing a marked increase activity at Axial Seamount with total seafloor uplift approaching the threshold depth for the 2015 eruption. As noted by W. Chadwick (OSU), whose website provides daily forecasts, the average differential inflation rate has almost doubled in the last six months. Bottom pressure tilt data at the Central and Eastern Caldera sites, show a marked increase in uplift beginning in April increasing from ~ 6 cm/yr to ~10 cm/year. The increase in uplift rates is coincident with a dramatic increase in seismic activity [viewable on daily plots of earthquakes accessible on W. Wilcock’s Axial Earthquake Catalogue (UW)] with >1000 earthquakes in a 24 hr period also occurring in April: seismic activity remains, high, but has not reached the 1000’s per day as detected prior to the April 2015 eruption (Wilcock et al, 2016). The hydrothermal system in the International District Hydrothermal Field, located on the eastern rift zone within Axial Caldera, is also responding to this increased activity. Fluid temperatures measured by the temperature-resistivity sensor in a parasitic orifice on the side of the hydrothermal vent Escargot show an increase in the past 6 months, with a marked change in the past 3 months (Courtesy of W. Ruef, UW). Excitement is building as we watch this dynamic volcano respond to melt migration 2 km below the seafloor – January 2025 is not far away.
[caption id="attachment_34576" align="alignnone" width="597"]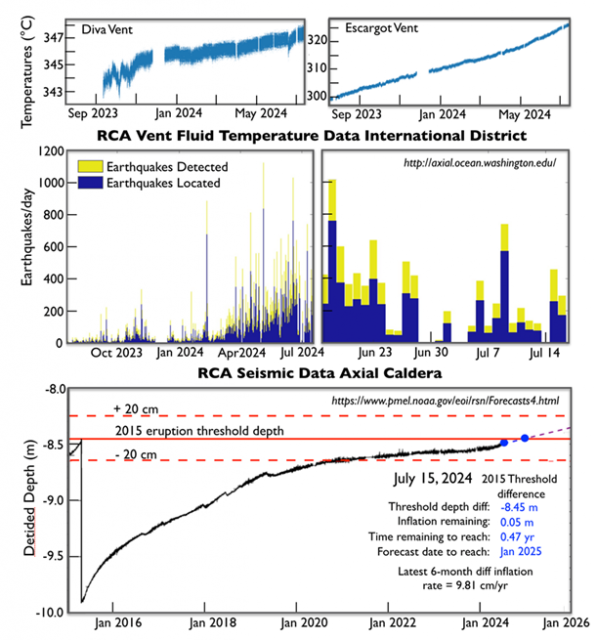
Widespread and Increasing Near-bottom Hypoxia in Pacific NW Coastal Ocean
Barth et al. (2024) examined the 2021 summer upwelling season off the United States Pacific Northwest coast. Upwelling was unusually strong leading to widespread near-bottom, low-oxygen waters. During summer 2021, an unprecedented number of ship- and underwater glider-based measurements of dissolved oxygen were made in this region. Near-bottom hypoxia, that is dissolved oxygen less than 61 µmol kg−1 and harmful to marine animals, was observed over nearly half of the continental shelf inshore of the 200-m isobath, covering 15,500 square kilometers. A mid-shelf ribbon with near-bottom, dissolved oxygen less than 50 µmol kg−1 extended for 450 km off north-central Oregon and Washington. Spatial patterns in near-bottom oxygen are related to the continental shelf width and other features of the region. Maps of near-bottom oxygen since 1950 show a consistent trend toward lower oxygen levels over time. The fraction of near-bottom water inshore of the 200-m isobath that is hypoxic on average during the summer upwelling season increases over time from nearly absent (2%) in 1950–1980, to 24% in 2009–2018, compared with 56% during the anomalously strong upwelling conditions in 2021. Widespread and increasing near-bottom hypoxia is consistent with increased upwelling-favorable wind forcing under climate change.
As part of their analysis, Barth et al. (2024) used NSF OOI glider data from 2021 along the Newport Hydrographic Line along with other data indicated in Fig x.. Near-bottom dissolved oxygen data from each survey as a function of time show the typical decrease of minimum values as the summer hypoxia season proceeds (Fig. x). High DO values are measured by the OOI gliders early in the upwelling season when winds were relaxed or downwelling-favorable early in the upwelling season, and by the Oregon Department of Fisheries and Wildlife (ODFW) survey that focused on very shallow (water depths of 50 m or less), inshore waters.
[media-caption path="https://oceanobservatories.org/wp-content/uploads/2024/05/Endurance-Figure.png" link="#"]Figure x Near-bottom dissolved oxygen as a function of time during the 2021 summer upwelling season.[/media-caption]__________________
Reference:
Barth, J.A., Pierce, S.D., Carter, B.R. et al. Widespread and increasing near-bottom hypoxia in the coastal ocean off the United States Pacific Northwest. Sci Rep 14, 3798 (2024). https://doi.org/10.1038/s41598-024-54476-0
Read MoreOOI Data Sonification
The assumption that quantitative data can be well represented not only in charts and graphs, but by increasingly sophisticated visual displays, is often taken for granted. However, researchers, instructors, and curators of museums and science centers also recognize that even the most sophisticated visual displays are inaccessible to those with low-vision or blindness. There is also the potential for alternative data presentation methods to improve understanding of complex data for sighted individuals. With these considerations in mind, a team led by Dr. Bower (WHOI) has pursued the creation of auditory displays or “data sonifications” using multi-disciplinary U.S. National Science Foundation Ocean Observatories Initiative (OOI) data sets as the basis. The results to date from the NSF-funded data sonification project are reported in a recent publication by Smith et al. (2024).
[media-caption path="https://oceanobservatories.org/wp-content/uploads/2024/05/Data-Sonification-figure.png" link="#"]Figure 1. Time series data from two data nuggets created by Ocean Data Labs that were used for sonification. Surface meteorology during the passage of hurricane Hermine over the Pioneer Array in 2016 (upper). The CO2 flux between ocean and atmosphere for both Pioneer (open circles) and Endurance Arrays during 2017 (lower).[/media-caption]Data sonification involves the mapping of quantitative data from its original form to audio signals in order to communicate complex information content. The project team was interested in using actual ocean data spanning a variety of oceanographic disciplines. Time series data produced by OOI sensors provide an excellent starting point. In particular, the Ocean Data Labs group at Rutgers has reviewed OOI data and created a set of “data nuggets” that are appropriate for sonification (Greengrove et al., 2020). The data nuggets comprise a broad range of oceanographic phenomena observed by OOI sensors, including response to a storm, the diurnal migration of zooplankton, a volcanic eruption, and the flux of CO2 between ocean and atmosphere.
The project team used a rigorous approach to developing and refining the auditory presentations. Starting with a set of learning objectives for each data nugget, a multi-step process was used to create the sonification. First, oceanographers were interviewed to establish the important points to be conveyed for a given data nugget. Next, classroom instructors were interviewed to get feedback on the most effective approaches to using sound to explain data properties. A sound designer then created an initial mapping of the data to sound, which was reviewed by a representative group of researchers, instructors, and blind and visually impaired listeners. After additional rounds of refinement and feedback, the prototype sonifications are now available. Two auditory displays utilizing Pioneer Array data (Fig. 1) are available at https://doi.org/10.5281/zenodo.8162769 and https://doi.org/10.5281/zenodo.8173880 for CO2 flux and storm response, respectively. Other examples can be found in Smith et al. (2024). The sonifications will be evaluated broadly using an on-line survey and by a “live audience” at museums and science centers.
This project is unique in exploiting the rich OOI data set and making ocean science highlights available to a broad community of students and the general public. A significant aspect of the work, as pointed out by the authors, is the systematic and inclusive approach used to develop the data sonifications. Results of the museum testing phase in 2024 will be awaited with great anticipation.
___________________
References:
Greengrove, C., S. Lichtenwalner, H.I. Palevsky, A. Pfeiffer-Herbert, S. Severmann, D. Soule, S. Murphy, L.M. Smith and K. Yarincik, 2020. Using authenticated data from NSF’s Ocean Observatories Initiative in undergraduate teaching, Oceanography, 33(1), 62-73.
Smith, L.M., A. Bower, J. Roberts, J. Bellona and J. Li, 2024. Expanding access to ocean science through inclusively designed data sonifications, Oceanography, 36(4), 96-101.
Read More
QARTOD Flag Statistics Using Cloud-Based Resources and Zarr Data Formats
Central to the Regional Cabled Array (RCA) Quality Assurance/Quality Control (QA/QC) workflow is a cloud-based data harvest and visualization platform that allows for efficient and timely review of the high-frequency and high-density time series data streaming from RCA instruments. A key component of this workflow is a Zarr-based version of the OOI RCA data, a cloud optimized file format that stores metadata separately, allowing data chunks to be retrieved without loading the entire dataset. Using Zarr files and Amazon Web Services (AWS) cloud computing resources for QA/QC has made it possible to create a dashboard that is refreshed daily for multiple high-density datasets and parameters. This also results in minimization of both costs and OOI-Cyberinfrastructure load by appending only new data daily, with a full dataset refresh occurring only when necessary. Because the Zarr files contain time-series data records that include all variables served through M2M (Machine to Machine Learning), this workflow also allows for the efficient deep data review that is currently required for compiling and reviewing QARTOD (Quality Assurance/Quality Control for Real-Time Oceanographic Data) data flag tables.
With the existing Zarr files, we were able to quickly access all active QARTOD flags in the RCA data streams for gross range and climatology and produce basic summary statistics for each parameter using minimal AWS cloud and data team resources. The figures above include the results from CTD (Conductivity, Depth, and Temperature) streams across the RCA for temperature and salinity, for both gross range and climatology QARTOD tests. In this initial compilation over 90% of all data points pass the gross range tests for temperature, and all except 2 pass gross range tests for salinity. Similar results were seen with the climatology tests, with nearly all streams having less than 10% of data points flagged as “suspect or interesting”. Most of the flags for non-passing data points in the climatology results were values of “not evaluated” in the Offshore and Slope Base Shallow Profiler streams. Our first set of investigations will focus on these streams to identify the cause for these flags. A suspected cause may be that the isoclines in the water column are not being accurately represented in the climatology depth bins.
Read More
Diel Vertical Migrators Respond to Short-Term Upwelling Events
Sato and Benoit-Bird, in their 2024 publication, explore how animals remain in a productive yet highly advective environment in the Northern California Current System using NSF OOI Regional Cabled Array (RCA) and Endurance Array (EA) data from the Oregon Shelf site. They characterized fish biomass using upward-looking active bio-acoustic sonar data from the RCA and interpreted results in consideration of upwelling and downwelling using EA wind data and combined cross-shelf velocity data from the RCA and EA.
Acoustic scatterers, consistent with swim bladder-bearing fish, were only present during the downwelling season as these animals avoided the cold waters associated with strong upwelling conditions in summer and fall. Fish responded to short-term upwelling events by increasing the frequency of diel vertical migration. Throughout the study, their vertical positions corresponded to the depth of minimum cross-shelf transport, providing a mechanism for retention. The observed behavioral response highlights the importance of studying ecological processes at short timescales and the ability of pelagic organisms to control their horizontal distributions through fine-tuned diel vertical migration in response to upwelling.
Time series data provided by the combined EA and RCA data made it possible for Sato and Benoit-Bird to perform consistent statistical analyses of bio-acoustic sonar, wind and ocean velocity data [Figure 22, after Figure 4, Sato and Benoit-Bird (2023)]. The vertical positions of scattering layers relative to the cross-shelf velocities revealed the careful positioning of animals at the depth of minimum onshore- offshore transport. The authors focused on cross-shelf transport, the most significant mechanism affecting population dynamics of pelagic organisms. During strong upwelling periods, cross-shelf velocities were strong near the surface and became nearly zero below 15-m depth. The peak scattering layers were in the upper 20 m of the water column during daytime, but organisms avoided the strong offshore currents at the surface (Figure 22a). At night, the scattering layers expanded their vertical distributions, but avoided the region nearest the bottom where onshore currents were strong (Figure 22b). During downwelling periods, scattering layers were located at the depth of minimal transport during day and night and animals avoided strong onshore currents near the surface and offshore currents near the bottom (Figures 22c and 22d).
[media-caption path="https://oceanobservatories.org/wp-content/uploads/2024/02/Science-Highlight-Feb-2024.jpg" link="#"]Figure 22: The influence of upwelling and downwelling on diel vertical migration[/media-caption]Sato and Benoit-Bird show that animals respond to the risk of offshore advection through active changes in their vertical movement that depend on upwelling conditions at daily time scales. Rapid behavioral response of animals to short-term upwelling events highlights their ability to finely tune their vertical positions relative to physical forcing which ultimately controls their horizontal distributions. This work expands our understanding of the ecological role of diel vertical migration beyond its role as a predator avoidance strategy and reveals a tight coupling between animal behavior and physical forcing.
Vertical profiles of cross-shelf velocities (u; gray, solid lines) and volume backscattering strength (Sv; colors, dotted lines), shown as mean ± standard deviations, during (a, b) strong upwelling periods with diel vertical migration and (c, d) strong downwelling periods without diel vertical migration. Data points qualified for strong upwelling and downwelling periods were selected from the time series over 14 months. Negative values in u indicate offshore transport and positive values indicate onshore transport, and larger Sv values suggest higher density of swim bladdered fish.
Read More